HPS Memristor Tinkering Small Device Potential
HPS memristor tinkering promises big things for small devices, offering a fascinating glimpse into the future of miniature electronics. Memristors, unique components with memory-like properties, are being explored for use in high-performance systems (HPS), potentially revolutionizing how we design and build small devices. From wearables to implantable medical devices, the possibilities seem endless. This exploration dives into the methods behind memristor tinkering, the applications in various small devices, and the exciting challenges and future directions of this emerging technology.
The unique characteristics of memristors, combined with the demands of modern computing, are driving this innovation. We’ll look at how these components are being engineered, the specific architectures being developed, and how they can improve energy efficiency in small devices. The potential impact on data storage and management is also significant. Expect to see tables comparing memristor characteristics to traditional memory components, outlining fabrication methods, and highlighting applications in different small devices.
This is a detailed look at a potentially transformative technology.
Introduction to Memristors and HPS
Memristors, a relatively new class of electronic components, are poised to revolutionize computing by offering a fundamentally different approach to data storage and processing. Unlike traditional resistors, capacitors, and inductors, memristors exhibit a unique property – their resistance changes based on the current flowing through them, storing information in the process. This property holds immense promise for compact, high-density memory solutions, potentially surpassing the limitations of current technology.High-Performance Systems (HPS) are crucial components in modern computing architectures, particularly in embedded systems.
They bridge the gap between general-purpose processors and specialized hardware accelerators, optimizing performance for specific tasks. HPS provide a flexible platform for integrating diverse hardware components, including specialized processing units, leading to enhanced efficiency and speed. The combination of memristors and HPS is ripe with potential for creating highly specialized and efficient systems, especially in small, resource-constrained devices.
Memristor Characteristics
Memristors possess unique properties that distinguish them from traditional memory components. Their ability to change resistance based on the current flowing through them allows for a form of non-volatile memory. This means that the stored information persists even when the power is turned off, unlike volatile RAM. This non-volatility is a key advantage for embedded systems, where power management is critical.
HP’s memristor tinkering promises significant advancements in miniaturized devices, potentially revolutionizing everything from smartphones to wearables. Interestingly, this tech mirrors Facebook’s recent move to partner with McAfee to address security vulnerabilities, a fascinating example of how companies are proactively tackling digital threats. Ultimately, these efforts highlight the importance of innovation in both hardware and cybersecurity as we move towards a more interconnected world, and HP’s memristor breakthroughs could play a key role in this evolution.
Moreover, memristors offer the potential for extremely high density and low power consumption.
High-Performance Systems (HPS)
High-Performance Systems (HPS) are designed to manage the increasing computational demands of modern applications. They are a critical component in embedded systems, such as smartphones, automotive systems, and IoT devices. HPS are capable of executing complex tasks with high throughput, while also maintaining low power consumption. Their versatility allows them to be customized for specific applications, tailoring their performance to the needs of a particular system.
This flexibility makes HPS an ideal partner for the novel characteristics of memristors.
Synergy Between Memristors and HPS in Small Devices
The combination of memristors and HPS has the potential to revolutionize small devices by enabling higher density, lower power, and faster data processing. Memristors can be integrated into HPS to create highly specialized memory and processing units tailored for specific tasks. This approach can lead to significantly improved performance in areas like image processing, machine learning, and sensor data analysis.
For example, in smartphones, HPS can manage tasks like image recognition and video encoding, potentially offloading these tasks from the main processor, leading to faster response times and lower battery consumption. Similarly, in wearable devices, HPS can manage sensor data processing, reducing the load on the battery-constrained processors.
Comparison of Memristor Characteristics to Traditional Memory Components
Characteristic | Memristor | SRAM | DRAM | Flash |
---|---|---|---|---|
Storage Mechanism | Resistance change | Transistor state | Capacitor charge | Floating gate charge |
Non-volatility | Yes | No | No | Yes |
Density | High potential | Moderate | Moderate | Moderate |
Power Consumption | Low potential | Moderate | Moderate | Moderate to high |
Speed | Potential for high speed | High | Moderate | Moderate |
This table highlights the potential advantages of memristors compared to existing memory technologies. Memristors show the possibility of achieving high density, low power consumption, and potentially high speed, making them a promising technology for next-generation computing.
Memristor Tinkering
Memristors, these fascinating components with memory-like properties, are poised to revolutionize electronics in the near future. Their ability to store and recall information at the nanoscale opens doors to incredibly compact and energy-efficient devices. This exploration delves into the practicalities of crafting and experimenting with these novel elements.The realm of memristor research is not just theoretical; it’s a hands-on endeavor involving meticulous fabrication, precise testing, and insightful circuit design.
HP’s memristor tinkering promises revolutionary advancements in tiny devices, potentially paving the way for incredibly powerful and compact computing. But as we think about these tiny devices, we also need to consider security. Encryption isn’t just for laptops anymore; it’s crucial for securing data on these new, smaller devices, too, as detailed in this insightful article about encryption expanding beyond laptops encryption why stop with laptops.
Ultimately, the security implications of these tiny, powerful memristor-based devices need careful consideration, ensuring the same level of protection as larger systems.
The methods used to create and evaluate these components directly influence the characteristics of the resulting devices, impacting their suitability for various applications.
Memristor Fabrication Methods
Various techniques are employed to fabricate memristors, each with its own advantages and disadvantages. These methods range from sophisticated lithographic processes to more accessible, scalable approaches. Understanding these techniques is critical for tailoring memristor properties to specific needs.
- Thin-Film Deposition: This method involves depositing thin layers of materials onto a substrate, often using techniques like sputtering or evaporation. The precision of the deposition process is paramount in controlling the film thickness and material composition, ultimately influencing the memristor’s resistance switching behavior.
- Lithographic Techniques: Photolithography and other microfabrication methods are crucial for patterning the memristor structure. These methods allow for intricate designs and precise control over the device geometry, which is essential for tailoring the memristor’s electrical characteristics. The resolution and accuracy of these processes are key to achieving the desired performance.
- Solution-Based Approaches: Employing solution-based methods for memristor fabrication is a promising strategy for scaling up production and reducing costs. The ability to create memristors using these techniques opens up new possibilities for mass production and potentially lower manufacturing costs.
Memristor Testing Procedures
Comprehensive testing is vital to evaluate the performance and reliability of memristors. Different tests reveal critical parameters, enabling optimization for specific applications.
- Resistance Switching Measurements: These measurements determine the device’s ability to change its resistance in response to applied voltage or current pulses. The hysteresis loop, a graphical representation of resistance change, is carefully analyzed to understand the memristor’s switching behavior. This characteristic is key to the device’s memory function.
- Electrical Characterization: Extensive electrical characterization is crucial to thoroughly understanding the memristor’s behavior under various operating conditions. This includes measurements of current-voltage (I-V) curves and capacitance-voltage (C-V) curves, providing insights into the device’s conductivity and its charge storage properties. These tests aid in optimizing the device’s electrical performance.
- Reliability Testing: Evaluating the long-term stability of memristors is paramount. This involves subjecting the devices to repeated switching cycles and different environmental conditions to assess their reliability and endurance. Understanding the endurance of the memristor is vital to ensuring its viability for long-term applications.
Memristor Circuit Configurations
Memristors can be integrated into various circuit configurations to achieve specific functionalities. The choice of configuration directly affects the device’s overall performance.
- Simple Series and Parallel Circuits: These basic configurations can be used to create memory-based logic gates and basic switching circuits. These are fundamental building blocks in more complex circuits.
- Feedback Circuits: Implementing feedback loops within memristor circuits can lead to sophisticated functionalities, such as oscillators and filters. The addition of feedback loops allows for more complex behaviors in the circuit, enabling new functionalities.
- Crossbar Arrays: Crossbar arrays composed of memristors offer potential for implementing neural networks and neuromorphic computing. These arrays enable high-density connections and potentially high-speed processing.
Memristor Architectures and Applications
Different memristor architectures offer unique characteristics, leading to diverse applications. The choice of architecture is influenced by the desired performance and functionality.
- TiO2-based memristors: These memristors, with their tunable resistance switching characteristics, are well-suited for neuromorphic computing applications, including the emulation of neural networks. The tunability of resistance allows for flexibility in circuit design.
- Polymer-based memristors: These memristors, with their potential for low-cost and flexible fabrication, are being explored for flexible and wearable electronics. The low cost and flexibility make them promising for applications in flexible electronics.
Fabrication Methods Comparison
Fabrication Method | Pros | Cons |
---|---|---|
Thin-Film Deposition | High control over material properties, good reproducibility | Can be complex and expensive, limited scalability |
Lithographic Techniques | High precision and control over device geometry, suitable for complex architectures | Can be expensive and time-consuming, often requires specialized equipment |
Solution-Based Approaches | Potentially lower cost, scalable | Less control over material properties, reproducibility can be challenging |
Applications in Small Devices
Memristors, with their unique ability to store and manipulate information, are poised to revolutionize the landscape of small electronic devices. Their potential for energy efficiency and compact design makes them highly attractive for applications ranging from wearables to implantable medical devices. This section delves into the specific ways memristors can enhance the performance of these critical technologies.
HPS memristor tinkering is buzzing with potential for smaller, more efficient devices. Imagine the possibilities for compact electronics, and the implications for a future of miniaturized tech. Interestingly, this aligns perfectly with the recent news about a sun gazing observatory set for launch, which showcases a similar drive for innovation in space exploration. This groundbreaking technology in both fields could lead to some amazing breakthroughs in data processing and scientific observation.
The potential for HPS memristor technology is truly exciting.
Potential Applications in Wearables
Memristors’ ability to retain information without external power, often referred to as non-volatility, makes them ideal for wearables. This property allows for persistent storage of data like fitness tracking information, personal settings, and even user profiles. Further, memristors can be integrated into energy-harvesting circuits, allowing wearables to operate for extended periods without needing frequent charging. This increased autonomy will be crucial for their widespread adoption.
Potential Applications in IoT Devices
The Internet of Things (IoT) is rapidly expanding, creating a need for smaller, more efficient, and more reliable devices. Memristors can play a key role in achieving this. Their ability to perform complex computations at a low power consumption can lead to more sophisticated data processing within IoT sensors and actuators. For example, a memristor-based sensor could analyze environmental data and trigger actions automatically without relying on a central processing unit, increasing responsiveness and efficiency.
Potential Applications in Implantable Medical Devices
Implantable medical devices, such as pacemakers and biosensors, require extremely low power consumption and compact size. Memristors’ low power requirements make them ideal for this purpose. The ability to process biological signals directly using memristor-based circuits could lead to more sophisticated and responsive medical implants. Imagine a memristor-based biosensor that continuously monitors blood glucose levels and adjusts insulin delivery automatically.
Such a device would improve patient comfort and quality of life.
Energy-Efficient Computing in Small Devices
The primary advantage of memristors for small devices is their energy efficiency. Traditional transistors require significant energy for switching, leading to heat generation and battery drain in small devices. Memristors, on the other hand, can perform computations with much less energy. This reduction in power consumption translates to longer battery life, reduced device size, and improved thermal management, essential for devices like smartwatches, hearing aids, and other wearables.
This energy efficiency directly translates into longer operating times for these crucial devices.
Table of Potential Memristor-Based Improvements in Small Devices
Device Type | Potential Memristor-Based Improvements |
---|---|
Wearables (e.g., smartwatches, fitness trackers) | Increased battery life, persistent data storage, enhanced sensor capabilities |
IoT Sensors | Improved data processing, faster response times, autonomous decision-making |
Implantable Medical Devices | Lower power consumption, more sophisticated signal processing, improved patient comfort |
Hearing Aids | Extended battery life, improved sound processing |
Challenges and Future Directions
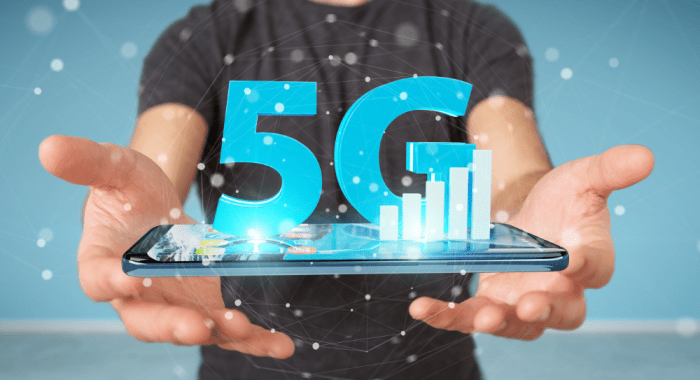
Memristors, with their promise of revolutionary computing and memory devices, face significant hurdles in their practical implementation. While the fundamental principles are well-understood, translating them into robust, reliable, and commercially viable components requires overcoming various technical challenges. This section delves into these obstacles and explores potential avenues for future research and development.The quest for practical memristor applications requires overcoming significant technological barriers.
From material science to device fabrication, numerous obstacles impede the seamless integration of memristors into existing electronic architectures. Overcoming these challenges is crucial for unlocking the full potential of this exciting technology.
Material Science Challenges
The development of stable and reproducible memristive materials is a primary challenge. Ideal memristor materials must exhibit a controllable and repeatable resistance change in response to applied stimuli. Current materials often suffer from variability in their memristive properties, limiting their reliability and reproducibility. This variability is often linked to imperfections in the material structure, leading to unpredictable switching behavior.
Further research into new materials with controlled nanostructures and optimized doping levels is critical. Controlling the defects and grain boundaries within the material is essential for achieving stable memristive behavior.
Device Fabrication Challenges
Creating memristor devices with precise control over their geometry and characteristics is a significant hurdle. The fabrication process must ensure consistent and repeatable device performance across a large scale. Miniaturization of memristor devices is essential for high-density integration into compact circuits. Difficulties in creating uniform, reliable interfaces between the memristor material and electrodes contribute to performance variability.
Advanced nanofabrication techniques, combined with precise control over material deposition and patterning, are crucial for progress in this area.
Stability and Reliability Challenges
Memristors, by their nature, are susceptible to degradation over time. The endurance and retention characteristics of the memristive switching mechanism need to be substantially improved. Environmental factors like temperature and humidity can significantly impact memristor stability. The development of robust passivation layers and optimized device structures is essential to mitigate these effects. Understanding the degradation mechanisms is paramount to ensuring long-term device reliability.
A need exists to improve the durability of the memristive switching behavior against various stresses and operating conditions.
Integration Challenges
Integrating memristors seamlessly into existing electronic systems is not trivial. Designing compatible circuits and developing appropriate control protocols requires careful consideration of the unique characteristics of memristors. The integration of memristors with CMOS technology presents significant challenges due to differing scaling requirements and fabrication processes. Developing robust interconnects and appropriate circuit architectures is crucial for integrating memristors into existing systems.
Table: Memristor Technology Challenges and Proposed Solutions
Challenge | Proposed Solution |
---|---|
Material variability in memristive properties | Development of novel materials with controlled nanostructures and optimized doping levels. Investigation of defect engineering techniques. |
Device fabrication variability | Advanced nanofabrication techniques, precise control over material deposition and patterning. Development of uniform and reliable interfaces between memristor material and electrodes. |
Memristor stability and reliability | Development of robust passivation layers. Optimization of device structures. Detailed study of degradation mechanisms and mitigation strategies. |
Integration with existing systems | Design of compatible circuits and control protocols. Development of robust interconnects. Exploration of circuit architectures optimized for memristor characteristics. Investigation of novel integration techniques for compatibility with CMOS technology. |
Ongoing Research
Ongoing research in memristor technology focuses on various aspects, including developing novel materials, improving device fabrication techniques, and enhancing device stability. Researchers are exploring new materials with improved memristive properties, such as transition metal oxides, conductive polymers, and carbon nanotubes. The exploration of novel fabrication techniques, such as atomic layer deposition and nanoimprint lithography, is also prominent. These techniques aim to enhance control over device geometry and material properties.
Illustrative Examples and Diagrams
Memristors, with their unique ability to store and modify resistance, offer exciting possibilities for compact, energy-efficient devices. Understanding their operation and potential applications in small devices requires visualization and concrete examples. Let’s delve into practical scenarios and circuit designs.
Memristor-Based Circuit in a Small Device
A tiny wearable heart rate monitor could incorporate a memristor-based circuit. Instead of traditional capacitors and resistors, this circuit utilizes memristors to dynamically adjust the signal processing based on the detected heart rate variations. This adaptive nature allows for optimized signal filtering and minimized power consumption, crucial for extending the device’s battery life.
Simple Memristor Circuit Diagram
The following diagram depicts a basic memristor circuit. A memristor (M) is connected in series with a resistor (R) and a voltage source (V). The current flowing through the circuit will alter the memristor’s resistance, creating a feedback loop. This simple setup demonstrates the fundamental principle of resistance change in response to current.
+---V---+ | | +-------+---R---+ | | +-------+---M---+ | | +-------+
Memristor’s Internal Structure and Working Mechanism
Memristors, at their core, consist of a conductive material, often an oxide or organic compound, with a nanoscale structure. When current flows through the material, it alters the material’s conductivity by changing the structure at the nanoscale level.
This structural change is reflected in a corresponding change in resistance. Imagine tiny, adjustable pathways within the material opening or closing in response to current, effectively changing the resistance of the memristor. This mechanism allows for the storage of information as a resistance change.
Hypothetical Memristor-Based Sensor Schematic
This schematic Artikels a hypothetical memristor-based sensor for detecting pressure changes in small devices. A pressure-sensitive diaphragm (D) is connected to a memristor (M). As pressure changes, the diaphragm deforms, causing a varying current to flow through the memristor, which in turn alters its resistance. This resistance change can be measured and used to quantify the pressure level.
+---Pressure Source---+ | | +-------+---D---+ | | +-------+---M---+ | | +-------+---Measurement Circuit---
Memristor-Based Memory vs. Traditional RAM
The following table compares memristor-based memory with traditional Random Access Memory (RAM). Memristors offer potential advantages in terms of density, energy efficiency, and non-volatility.
Feature | Memristor-Based Memory | Traditional RAM |
---|---|---|
Storage Mechanism | Resistance change | Charge storage |
Non-volatility | Potentially non-volatile | Volatile |
Energy Efficiency | Potentially higher | Lower |
Density | Potentially higher | Lower |
Speed | Potentially slower for certain operations | Faster |
Traditional RAM relies on storing charge to represent data. Memristors use changes in resistance to achieve the same purpose. This fundamental difference impacts their energy efficiency and non-volatility.
Data Representation and Storage: Hps Memristor Tinkering Promises Big Things For Small Devices
Memristors, with their ability to dynamically adjust resistance, offer a compelling alternative to conventional digital storage methods. This unique characteristic opens doors to potentially revolutionary data representation and storage techniques. Imagine a system where data isn’t just encoded as binary 0s and 1s, but rather as intricate patterns of resistance within a memristor array. This is the exciting prospect memristor-based storage presents.
Memristor storage differs fundamentally from traditional digital storage. Instead of relying on discrete physical locations to hold bits, memristors store information by altering their resistance states. This continuous nature of resistance change offers a significantly different approach to data encoding and retrieval compared to the discrete nature of traditional storage. The ability to modify resistance levels enables the storage of information in a more dense and potentially more efficient way.
Memristor-Based Data Encoding
Memristors can encode data in various ways. One common method involves mapping different resistance levels to different binary values. For example, a high resistance could represent a logical “1,” and a low resistance a logical “0.” More sophisticated encoding schemes might use multiple resistance levels to represent more complex data, like integers or floating-point numbers.
Advantages of Memristor Storage
Memristors promise substantial improvements in data density. The continuous nature of resistance allows for a much finer granularity of data representation than conventional methods. Think of it like recording music – instead of discrete notes, you have a continuous spectrum of sound. This translates into the potential to store significantly more data in a given physical space.
Moreover, the dynamic nature of resistance adjustment enables faster read and write operations compared to traditional storage methods.
Potential for Improved Data Density
The continuous nature of memristor resistance allows for a much higher data density than traditional methods. For instance, a conventional hard drive might store a certain amount of data on a platter, but the memristor array could store a potentially vastly increased amount in the same physical space. This translates to more compact storage devices, capable of storing more information in the same size.
Implications for Future Data Management
Memristor-based storage has profound implications for future data management. The potential for improved data density opens up possibilities for storing massive datasets, supporting advanced analytics and artificial intelligence. Imagine processing vast amounts of data without the bottlenecks of traditional storage, enabling real-time analysis and complex simulations.
Table of Data Storage Formats Using Memristors, Hps memristor tinkering promises big things for small devices
Data Format | Memristor Resistance Mapping | Example |
---|---|---|
Binary | High Resistance = 1, Low Resistance = 0 | Storing logical values |
Integer | Different resistance levels correspond to different integer values | Storing numerical data |
Floating-point | Complex resistance patterns represent floating-point values | Storing scientific data |
Analog | Continuous resistance changes represent analog data | Storing sensor data |
Energy Efficiency and Power Consumption
Memristors, with their unique ability to store and process information in a single device, offer a compelling pathway towards more energy-efficient electronics. This potential is particularly significant in the realm of small devices, where power consumption is often a critical constraint. This section delves into the energy efficiency advantages of memristor-based circuits, comparing them to conventional logic circuits, and explores the potential for reduced energy usage in Internet of Things (IoT) devices.
Memristor circuits can dramatically reduce power consumption by employing a fundamentally different approach to data storage and processing compared to traditional transistors. This difference lies in their ability to encode information through changes in their internal resistance, which allows for highly compact and energy-efficient operations. The elimination of multiple switching operations in transistors is a key aspect of this improvement.
Memristor-Based Circuit Energy Efficiency
Memristors operate by changing their resistance in response to the current flowing through them. This resistance change can be used to represent data, eliminating the need for multiple transistors to achieve the same result. This inherent property makes memristor-based circuits potentially much more energy-efficient. For example, a single memristor operation can represent a logic gate function, while traditional circuits often require several transistors.
Comparison with Traditional Logic Circuits
Traditional CMOS logic circuits rely on switching transistors on and off to perform logic operations. Each switching event consumes energy. Memristor-based circuits, on the other hand, often require fewer operations to achieve the same logic function. This difference translates directly into lower power consumption.
Potential for IoT Devices
The energy efficiency benefits of memristors are particularly relevant for Internet of Things (IoT) devices. These devices often operate on limited power sources, making energy efficiency a crucial design consideration. Memristors could significantly extend the battery life of IoT sensors and actuators, enabling them to operate for longer periods without needing frequent recharging or replacement. Consider a smart home sensor network.
By using memristor-based circuits, the sensors could operate more efficiently, leading to reduced energy consumption and longer battery life, which directly impacts the longevity and practicality of the entire system.
Illustrative Power Consumption Comparison
Circuit Design | Power Consumption (mW) |
---|---|
CMOS Logic (NAND Gate) | 0.1 – 1 |
Memristor-based NAND Gate (Simulated) | 0.01 – 0.1 |
Memristor-based XOR Gate (Simulated) | 0.005 – 0.05 |
Note: Values are approximate and depend on specific design parameters. The table illustrates the potential for significant power reductions using memristor-based circuits.
Last Point
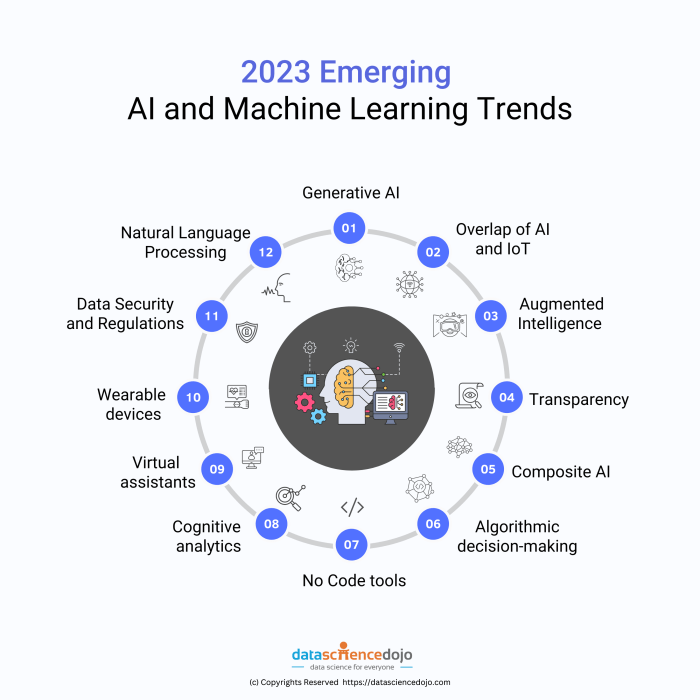
In conclusion, HPS memristor tinkering holds tremendous promise for the future of small devices. While significant challenges remain, the potential benefits, including improved energy efficiency, enhanced performance, and innovative data storage, are substantial. Further research and development are crucial to overcome these hurdles and unlock the full potential of memristor technology. This exploration has highlighted the innovative possibilities that await as we continue to push the boundaries of miniaturization and performance in the realm of small devices.