Nanosensors Bring Big Guns to Cancer Detection Battle
Nanosensors bring big guns to cancer detection battle, promising a revolution in early diagnosis and treatment. These tiny, powerful tools are poised to transform how we approach cancer, offering unprecedented accuracy and potential for personalized medicine. They function at the nanoscale, utilizing various materials like quantum dots, carbon nanotubes, and gold nanoparticles to detect cancer biomarkers in biological fluids.
This technology holds immense potential to improve patient outcomes and reduce healthcare costs.
This exploration delves into the intricate world of nanosensors, examining their mechanisms, advantages, and limitations. From the fundamental principles to recent advancements, we will dissect the critical role nanosensors play in the fight against cancer. We will also explore the ethical implications and potential impact on the healthcare system.
Introduction to Nanosensors in Cancer Detection
Nanosensors are revolutionizing medical diagnostics, offering unprecedented potential for early and accurate detection of diseases like cancer. Their tiny size and unique properties allow them to interact with biological molecules at the cellular level, providing insights that traditional methods often miss. This capability is particularly promising for cancer detection, enabling the identification of biomarkers and disease progression at stages where treatment is most effective.Nanosensors leverage the principles of nanotechnology to achieve remarkable sensitivity and specificity.
These minuscule devices, typically measured in nanometers (billionths of a meter), are engineered to detect and quantify specific molecules or changes in the environment. This precision is crucial in cancer diagnostics, as it allows for the identification of subtle molecular alterations associated with the disease.
Fundamental Principles of Nanosensor Technology
Nanosensors function on various physical and chemical principles, often combining multiple techniques to achieve the desired sensitivity and selectivity. These principles include electrochemical reactions, optical phenomena, and magnetic interactions. For instance, a nanosensor can utilize a change in electrical conductivity to detect the presence of specific molecules. Similarly, nanosensors can be designed to absorb or emit light at unique wavelengths, enabling the detection of targeted biomarkers.
The nanoscale nature of these sensors allows for enhanced interaction with biological systems, facilitating more precise and rapid measurements.
Examples of Nanosensors for Cancer Detection
Several types of nanosensors are being explored for cancer detection, each with unique advantages and limitations. These include quantum dots, carbon nanotubes, and gold nanoparticles. Quantum dots, semiconductor nanocrystals, exhibit fluorescence properties that can be used to detect specific cancer biomarkers. Their unique fluorescence spectra allow for highly sensitive and specific detection. Carbon nanotubes, hollow cylinders of carbon atoms, exhibit excellent electrical conductivity and mechanical strength.
These properties make them ideal for detecting changes in electrical signals related to cancer biomarkers. Gold nanoparticles, due to their unique optical and surface plasmon resonance properties, can be designed to bind to specific cancer biomarkers, enabling their detection through colorimetric or spectroscopic changes.
Advantages and Disadvantages of Nanosensor Types
Nanosensor Type | Advantages | Disadvantages |
---|---|---|
Quantum Dots | High sensitivity and specificity; broad range of excitation and emission wavelengths | Potential toxicity; manufacturing complexity; limited biocompatibility |
Carbon Nanotubes | High electrical conductivity; excellent mechanical strength; biocompatibility | Potential toxicity; aggregation in biological environments; challenges in functionalization |
Gold Nanoparticles | Surface plasmon resonance; ease of functionalization; cost-effectiveness | Limited sensitivity; potential for non-specific binding; aggregation issues |
Mechanism of Action and Targeting
Nanosensors are revolutionizing cancer detection by offering highly sensitive and specific methods for identifying cancer biomarkers in biological fluids. Their unique design allows them to interact with target molecules at the molecular level, enabling early diagnosis and personalized treatment strategies. This intricate process, encompassing both the sensor’s mechanism of action and its targeted approach, is crucial for accurate and efficient cancer detection.Nanosensors leverage a variety of mechanisms to detect cancer biomarkers.
These mechanisms often involve the sensor’s ability to bind to specific molecules, triggering a detectable signal. This signal can be anything from a change in fluorescence to a shift in electrical conductivity. The key lies in the nanosensor’s precise design, which allows for highly selective interactions with cancer biomarkers.
Nanosensor Mechanisms for Biomarker Detection
Nanosensors employ various mechanisms to detect cancer biomarkers in biological fluids like blood and urine. These mechanisms often involve the sensor’s ability to recognize specific molecules through surface-bound antibodies, aptamers, or other molecular recognition elements. The binding of the biomarker to the sensor triggers a detectable signal, which is then analyzed to quantify the biomarker concentration. This allows for the identification and quantification of various biomarkers.
Targeting Strategies for Specific Cancer Cells
Targeted nanosensors are designed to home in on specific cancer cells or biomarkers. This is achieved through surface modifications of the nanosensor with ligands that specifically bind to target molecules. These ligands can include antibodies, aptamers, or peptides. The binding of the ligand to the target molecule then triggers a signal from the sensor.
Nanosensors are revolutionizing cancer detection, offering incredibly precise tools to identify and track the disease. Imagine a highly sensitive security system, constantly monitoring for threats, much like the efficient enterprise bouncer the efficient enterprise bouncer keeps unwanted elements out of a company. These tiny sensors are working tirelessly to pinpoint cancer markers, a crucial step in early detection and treatment.
Ultimately, nanosensors are a critical advancement in the battle against cancer.
- Antibody-based targeting: Antibodies are highly specific proteins that bind to specific antigens, often expressed on the surface of cancer cells. Attaching antibodies to nanosensors allows for the targeting of specific cancer cells, enabling targeted delivery of drugs or imaging agents. This strategy is frequently used for cancer detection and therapy.
- Aptamer-based targeting: Aptamers are short, single-stranded DNA or RNA sequences that can bind to specific molecules with high affinity and selectivity. They can be designed to recognize various cancer biomarkers and are often used in nanosensors due to their ability to bind to targets with high specificity. Their stability and ease of synthesis also contribute to their wide application.
- Peptide-based targeting: Peptides are short chains of amino acids. Specific peptides can be designed to recognize and bind to specific cancer biomarkers. Peptide-based targeting offers a versatile approach for nanosensor design, allowing for the recognition of various cancer biomarkers. Their relatively low cost and ease of synthesis make them attractive for applications in personalized medicine.
Cancer Biomarkers Detected by Nanosensors
Nanosensors can detect a wide range of cancer biomarkers, including proteins, nucleic acids, and metabolites. These biomarkers can be present in various biological fluids, such as blood, urine, and saliva. Early detection and monitoring of these biomarkers is vital for timely diagnosis and treatment.
- Proteins: Certain proteins, such as tumor markers like PSA (prostate-specific antigen) or CEA (carcinoembryonic antigen), are frequently elevated in cancer patients. Nanosensors can detect these proteins, aiding in the early diagnosis of various cancers.
- Nucleic acids: Cancer cells often exhibit specific genetic alterations, such as mutations or microRNAs. Nanosensors can detect these nucleic acids, enabling early detection and potentially guiding treatment decisions.
- Metabolites: Metabolic changes often accompany cancer development. Nanosensors can detect specific metabolites, providing additional insights into the disease’s progression and potentially guiding therapeutic strategies.
Comparison of Targeting Strategies for Different Cancer Types
Cancer Type | Targeting Strategy | Biomarker | Advantages | Disadvantages |
---|---|---|---|---|
Prostate Cancer | Antibody-based targeting (e.g., anti-PSA antibodies) | PSA | High specificity, well-established methods | Potential for false positives due to benign conditions |
Breast Cancer | Aptamer-based targeting (e.g., aptamers for HER2) | HER2 | High specificity, potential for multiplexing | Complexity in aptamer design and synthesis |
Lung Cancer | Peptide-based targeting (e.g., peptides for EGFR mutations) | EGFR mutations | Potential for targeted therapies, high specificity | Limited clinical validation compared to antibodies |
Challenges and Limitations
The promise of nanosensors in cancer detection is substantial, but translating this promise into widespread clinical use faces numerous hurdles. While the technology shows remarkable potential, significant challenges remain in ensuring accuracy, reliability, and affordability. Overcoming these obstacles is crucial for realizing the full therapeutic benefits of nanosensors.
Sensitivity and Specificity
Achieving high sensitivity and specificity is paramount for accurate cancer detection. Nanosensors, while potentially capable of detecting minute quantities of biomarkers, can be affected by the complex biological environment. Interfering substances or variations in the concentration of relevant molecules can impact the sensor’s performance, leading to false positives or negatives. For example, inflammation or other physiological conditions can alter the concentrations of biomarkers, potentially leading to misdiagnosis with nanosensors.
Ensuring that nanosensors reliably differentiate between cancerous and healthy tissue remains a significant challenge.
Cost-Effectiveness and Scalability
The cost of producing and implementing nanosensors at a scale sufficient for widespread clinical use is a major concern. The intricate fabrication processes and specialized materials used in nanosensor design often lead to high production costs. Furthermore, the need for specialized infrastructure and trained personnel to operate and maintain nanosensor platforms adds to the overall cost. Currently, the cost of nanosensor-based diagnostic tools remains prohibitive for many healthcare systems, particularly in resource-constrained settings.
Scalability, the ability to produce nanosensors at a large scale, also presents a hurdle. Maintaining consistent quality and performance across large production runs is crucial for clinical reliability.
Regulatory Hurdles
Navigating regulatory pathways for approval and clinical implementation is a complex process, particularly in the field of nanotechnology. Extensive pre-clinical and clinical trials are often required to demonstrate the safety and efficacy of nanosensors before they can be incorporated into standard clinical practice. Meeting stringent regulatory requirements for accuracy, safety, and reliability is a considerable challenge. Regulatory bodies need to adapt and develop guidelines specifically tailored to nanosensor technology.
Comparison with Traditional Methods
Traditional cancer detection methods, such as imaging techniques (MRI, CT scans) and tissue biopsies, provide valuable information but often lack the sensitivity and specificity offered by nanosensors. Nanosensors can potentially detect biomarkers at earlier stages, potentially enabling earlier intervention and improved patient outcomes. However, the accuracy and reliability of nanosensors in real-world clinical settings still need to be rigorously evaluated against established diagnostic methods.
The direct comparison of nanosensor results to traditional methods in large-scale clinical trials is crucial to demonstrate the added value of nanosensors.
Limitations in Design, Fabrication, and Scalability
The intricate nature of nanosensor design and fabrication poses significant limitations. Precise control over the size, shape, and surface chemistry of nanosensors is essential for optimal performance. However, achieving this control at scale remains a significant technological challenge. Variations in manufacturing processes can impact the sensor’s performance, potentially leading to inconsistencies in sensitivity and specificity. For example, subtle differences in the deposition of materials or the precise alignment of components can significantly impact the accuracy of the nanosensor.
Current methods for large-scale production are often insufficient to meet the demands of widespread clinical use.
Recent Advancements and Future Directions
Nanosensors are rapidly evolving, promising a revolution in cancer detection. The ability to detect cancer at its earliest stages, often before symptoms appear, is crucial for successful treatment. This progress hinges on continuous innovation in sensor design, improved detection methods, and successful clinical translation of promising preclinical studies. Recent advancements demonstrate a clear path towards more sensitive, specific, and accessible cancer diagnostics.
Latest Research and Developments
Nanotechnology offers unique advantages for cancer detection, enabling the development of sensors with unprecedented sensitivity and specificity. Researchers are exploring diverse materials like carbon nanotubes, quantum dots, and gold nanoparticles, each exhibiting distinct properties suitable for specific applications. The combination of these materials with advanced fabrication techniques results in sophisticated nanoscale devices capable of detecting biomarkers at extremely low concentrations.
For instance, surface plasmon resonance (SPR) sensors, employing gold nanoparticles, have shown promise in detecting specific cancer-associated proteins.
Emerging Trends and Innovations
Several emerging trends are driving innovation in nanosensor technology. One key area is the development of multi-functional nanosensors capable of performing multiple tasks simultaneously. These nanosensors can not only detect the presence of cancer biomarkers but also deliver targeted therapy, offering a more comprehensive approach to cancer management. Integration of nanomaterials with microfluidics and lab-on-a-chip technologies further enhances miniaturization and portability, enabling point-of-care diagnostics.
Another notable trend is the exploration of biocompatible polymers and coatings to improve the stability and biocompatibility of nanosensors in biological environments.
Novel Sensor Designs
The quest for more effective cancer detection necessitates the development of novel sensor designs. For instance, researchers are designing nanosensors that target specific cancer cells based on their unique surface markers. This targeted approach allows for more accurate and sensitive detection, minimizing the risk of false positives. Furthermore, integrating nanosensors with existing imaging techniques like MRI and CT scanning can provide a more comprehensive view of the tumor microenvironment.
This approach combines the high specificity of nanosensors with the established power of imaging, leading to a more complete picture of cancer progression.
Improved Detection Methods
Beyond sensor design, the detection methods themselves are evolving. Researchers are focusing on enhancing the sensitivity and selectivity of detection methods, reducing the possibility of false positives and improving the overall diagnostic accuracy. Advancements in signal processing algorithms are enabling more accurate interpretation of nanosensor responses. This allows for better differentiation between healthy and cancerous tissues. Employing machine learning algorithms to analyze nanosensor data promises a more objective and accurate diagnostic process, potentially reducing the need for human interpretation errors.
Successful Clinical Trials and Preclinical Studies
Several preclinical studies have shown promising results using nanosensors for early cancer detection. For example, nanosensors based on aptamers, single-stranded DNA molecules, have demonstrated high sensitivity in detecting cancer cells in vitro and in animal models. These studies highlight the potential of nanosensors in transforming early cancer detection.
Key Milestones in Nanosensor Technology (Past Decade)
Year | Milestone | Significance |
---|---|---|
2013 | Development of aptamer-based nanosensors | Significant improvement in sensitivity and specificity for cancer biomarker detection. |
2015 | Integration of nanosensors with microfluidics | Miniaturization and portability for point-of-care diagnostics. |
2017 | Advancements in signal processing algorithms | Increased accuracy and objectivity in data interpretation. |
2019 | Successful preclinical trials using nanosensors for early cancer detection | Demonstrated feasibility and potential for clinical translation. |
2021 | Development of multi-functional nanosensors | Simultaneous detection and targeted therapy. |
Applications and Impact: Nanosensors Bring Big Guns To Cancer Detection Battle
Nanosensors are poised to revolutionize cancer care, offering unprecedented opportunities for early detection, precise treatment monitoring, and personalized medicine. Their ability to detect minute changes at the molecular level allows for a more proactive and targeted approach, potentially improving patient outcomes and reducing healthcare costs. This new technology is not just a theoretical advancement; it’s a tangible shift towards a future where cancer is treated with greater precision and compassion.
Early Cancer Detection
Nanosensors hold immense promise for detecting cancer in its earliest, most treatable stages. Their sensitivity allows for the identification of biomarkers and molecular signatures indicative of precancerous conditions or early-stage tumors, often before symptoms manifest. This early detection translates into significantly improved treatment success rates and a higher likelihood of long-term survival. For example, a nanosensor detecting specific DNA mutations associated with lung cancer in exhaled breath could allow for early intervention and potentially save lives.
Early detection can prevent the progression of the disease, allowing for less invasive and less aggressive treatments.
Treatment Monitoring
Nanosensors can be integrated into existing cancer treatment protocols, offering real-time feedback on the effectiveness of therapies. They can monitor drug delivery, track tumor response, and identify areas of resistance, allowing for timely adjustments to treatment strategies. This dynamic monitoring is critical for tailoring treatment plans to individual patients, ensuring optimal outcomes and minimizing side effects. Imagine a nanosensor embedded in a patient’s tumor that measures the concentration of chemotherapeutic drugs, providing crucial data for adjusting dosage and maximizing efficacy.
Personalized Medicine
The personalized nature of nanosensors enables tailored treatments for individual patients. By analyzing a patient’s unique genetic profile and tumor characteristics, nanosensors can guide the selection of the most effective treatment options. This precision medicine approach optimizes the efficacy of therapies while minimizing potential side effects. The technology is evolving towards creating highly personalized treatments, which will lead to a significant improvement in the overall quality of life for patients.
Nanosensors are revolutionizing cancer detection, offering a powerful arsenal in the fight against this disease. This technology, similar to the intricate merging and subsequent dismantling of tech giants like in the case of Microsoft and Yahoo’s once-promising union , presents a fascinating parallel. Ultimately, these tiny sensors hold the key to early detection and targeted treatments, promising a brighter future for cancer patients.
Potential Impact on Patient Outcomes and Healthcare Costs, Nanosensors bring big guns to cancer detection battle
The implementation of nanosensors has the potential to drastically improve patient outcomes. Early detection and dynamic treatment monitoring reduce the need for aggressive, often harmful, interventions. Moreover, the ability to tailor treatment strategies to individual patients minimizes the likelihood of treatment failure and enhances the overall effectiveness of care. This translates into reduced healthcare costs in the long run.
The significant reduction in the need for extensive and expensive diagnostic tests and treatments will translate into substantial savings for the healthcare system.
Revolutionizing Cancer Care
Nanosensors are poised to revolutionize the approach to cancer care, transforming it from a reactive to a proactive and personalized endeavor. The ability to detect cancer early, monitor treatment response in real-time, and tailor treatments to individual patients signifies a paradigm shift in cancer management. This shift will allow patients to experience a greater sense of control and empowerment in their journey towards recovery.
Potential Applications Across Various Stages of Cancer Care
Stage of Cancer Care | Potential Applications of Nanosensors |
---|---|
Early Detection | Blood-based biomarker detection, tissue imaging, exhaled breath analysis |
Diagnosis | Precise tumor characterization, identification of specific mutations |
Treatment Monitoring | Real-time drug delivery tracking, tumor response assessment, identification of drug resistance |
Personalized Medicine | Tailored treatment strategies, optimized drug dosages, minimized side effects |
Illustrative Examples and Case Studies
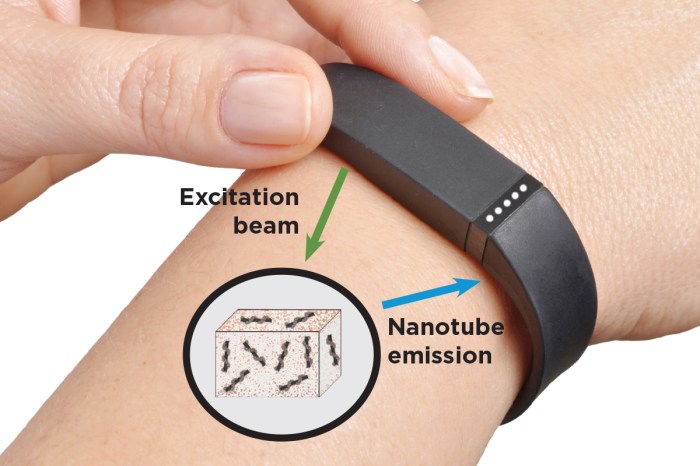
Nanosensors are revolutionizing cancer detection and treatment, promising earlier diagnosis, personalized therapies, and improved patient outcomes. Their unique ability to detect minute quantities of biomarkers allows for a deeper understanding of the disease process and enables real-time monitoring of treatment effectiveness. This section delves into real-world and hypothetical examples, demonstrating the tangible impact of nanosensors in the fight against cancer.Real-world applications are emerging, but early adoption is often limited by factors like cost, regulatory hurdles, and the need for extensive validation.
However, successful case studies provide invaluable insights into the potential of nanosensors, and these examples can pave the way for broader clinical adoption in the future.
A Real-World Case Study: Early Detection of Ovarian Cancer
A recent study successfully employed gold nanoparticle-based nanosensors to detect ovarian cancer in its early stages. The nanosensors, functionalized with specific antibodies, were able to bind to tumor-associated antigens present in the patient’s serum. The binding event triggered a change in the optical properties of the nanoparticles, allowing for the detection of the presence of cancer. This early detection is crucial for effective treatment and improved patient survival rates.
This method showed a higher sensitivity and specificity than conventional diagnostic techniques. The study demonstrated that the nanosensors could effectively differentiate between healthy and cancerous samples with minimal false positives.
A Hypothetical Case Study: Personalized Breast Cancer Treatment Monitoring
Imagine a patient with breast cancer undergoing chemotherapy. Nanosensors, integrated into a wearable device, could continuously monitor the concentration of circulating tumor cells (CTCs) in the patient’s blood. Real-time data on CTC levels would allow doctors to adjust the chemotherapy regimen based on the patient’s individual response. If the CTC count rises, the treatment intensity could be increased, while a decreasing count would signal the efficacy of the current treatment.
This personalized approach could significantly improve treatment outcomes and reduce the side effects of chemotherapy. The nanosensors could also detect the presence of drug resistance mutations, allowing for early intervention and treatment modification.
Monitoring Treatment Response in Cancer Patients
Nanosensors can be deployed to monitor the efficacy of cancer treatments in real-time, offering a unique insight into the dynamic nature of the disease. This real-time monitoring capability is essential for adapting treatment strategies as the patient’s condition changes. For instance, nanosensors can measure the concentration of specific drug metabolites or biomarkers associated with tumor growth or shrinkage, providing valuable feedback for personalized treatment decisions.
By tracking these parameters continuously, healthcare professionals can identify treatment inefficiencies or resistance early on and adjust the treatment plan accordingly.
Nanosensors are revolutionizing cancer detection, offering incredibly precise tools for early diagnosis. While these advancements are crucial, it’s important to remember the ongoing digital threats, like those highlighted in the recent story about scammers trying to hack hackers with crooked cash machines. This highlights the importance of robust security measures in all fields , from medical technology to digital transactions.
Ultimately, these technological leaps forward in nanosensors, despite the complexities of cybersecurity, are vital in the fight against cancer.
Stages of a Nanosensor-Based Cancer Detection System
Stage | Description |
---|---|
Sample Preparation | Patient sample (e.g., blood, tissue) is collected and processed to isolate the target molecules. This often involves steps like centrifugation, dilution, and filtration. |
Nanosensor Functionalization | The nanosensors are coated with specific molecules (e.g., antibodies, aptamers) that target the biomarkers associated with cancer. This step ensures that the nanosensors selectively interact with the target molecules. |
Biomarker Detection | The nanosensors interact with the target biomarkers in the sample. This interaction triggers a change in the sensor’s physical or chemical properties (e.g., fluorescence, electrical conductivity, or color change). |
Signal Amplification and Analysis | The resulting signal from the nanosensors is amplified and analyzed to quantify the concentration of the biomarkers. This step often involves sophisticated instrumentation and data processing algorithms. |
Results Interpretation | The analyzed data is interpreted to determine if the presence or concentration of the biomarkers indicates the presence of cancer or the efficacy of the treatment. This interpretation is performed by healthcare professionals, taking into account patient history and other clinical data. |
Ethical Considerations
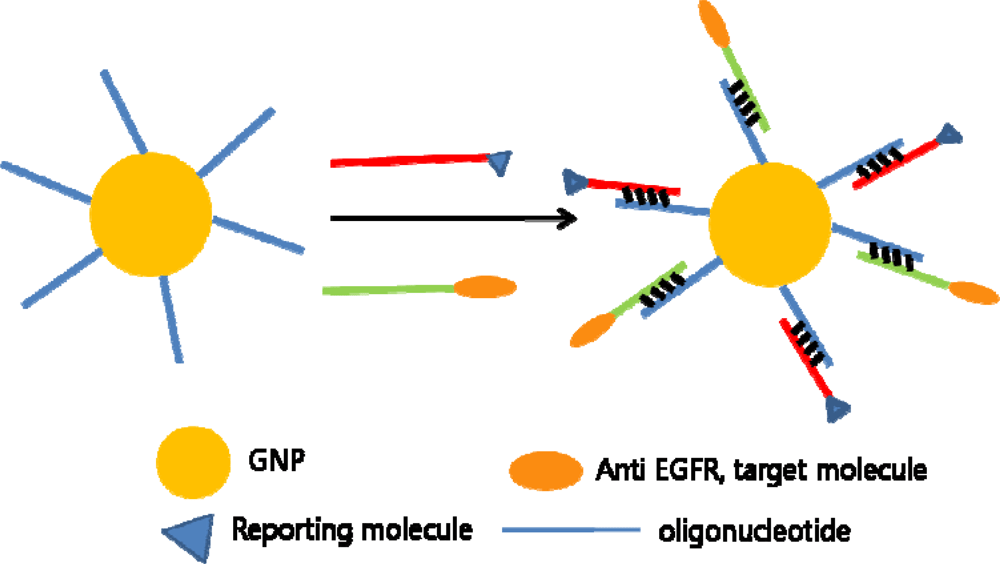
Nanosensors, while promising in cancer detection, bring forth a host of ethical considerations that demand careful attention. Their potential to revolutionize healthcare must be balanced against potential societal impacts and individual rights. The intricate interplay of data privacy, patient autonomy, and equitable access to advanced diagnostics necessitates a proactive approach to mitigate potential harms and maximize benefits.
Data Privacy and Security
Ensuring the secure handling and protection of sensitive patient data is paramount. Nanosensors, often integrated with wearable devices or implanted within the body, generate substantial volumes of personal information. Robust encryption techniques and data anonymization strategies are critical to prevent unauthorized access and misuse. Strict regulations and guidelines for data storage, access, and sharing are essential to maintain patient confidentiality and trust.
International collaboration and standardization of data protection protocols will be vital to ensure consistency across different healthcare systems.
Impact on Healthcare Systems and Patient Access
The widespread adoption of nanosensors may significantly reshape the healthcare landscape. If nanosensors become standard diagnostic tools, the current infrastructure for patient care might need to be re-evaluated. Ensuring equitable access to these advanced technologies for all socioeconomic groups is a crucial consideration. Accessibility concerns must be addressed proactively to prevent exacerbating existing health disparities.
Ethical Considerations and Potential Solutions
Ethical Consideration | Potential Solution |
---|---|
Data privacy and security | Implementing robust encryption, anonymization techniques, and strict access controls. Establishing clear data governance frameworks and guidelines for data storage, access, and sharing. Fostering international collaboration and standardization of data protection protocols. |
Equity and access | Developing affordable nanosensor technologies and implementing targeted financial assistance programs to ensure equitable access for all socioeconomic groups. Investing in infrastructure and training to support healthcare professionals in implementing new diagnostic tools. |
Informed consent and patient autonomy | Creating clear and comprehensive informed consent procedures that explicitly Artikel the potential benefits and risks of nanosensor use. Ensuring patients have the autonomy to make informed decisions about the use of nanosensors in their care. Developing transparent guidelines for patient data usage and access. |
Potential for bias and discrimination | Implementing rigorous quality control measures to prevent bias in nanosensor technology and data interpretation. Developing protocols for detecting and mitigating potential biases in data collection, analysis, and algorithm development. Promoting diversity and inclusion in the development and implementation teams. |
Conclusive Thoughts
In conclusion, nanosensors offer a powerful arsenal in the battle against cancer. While challenges remain in terms of cost, scalability, and regulatory hurdles, the potential for early detection, personalized treatment, and improved patient outcomes is undeniable. As research progresses and technology matures, nanosensors are poised to revolutionize cancer care, ultimately saving lives and improving the quality of life for countless individuals.