Tiny Nanoburrs Arterial Repair
Tiny nanoburrs stick to damaged arteries and repair tissue, offering a revolutionary approach to cardiovascular care. Imagine minuscule, specialized structures that latch onto damaged arterial walls, effectively patching the injury and promoting healing. This innovative technology leverages the principles of nanotechnology to tackle a critical medical challenge, offering potential solutions to cardiovascular diseases. The process involves various stages, from initial nanoburr design to the complex biological interactions that facilitate tissue repair.
This article delves into the intricacies of this promising technology, exploring its mechanisms, applications, and potential benefits.
The technology behind nanoburrs is built upon a foundation of nanomaterials, offering unique properties and functionalities. The fundamental principles of their interaction with damaged tissue are explored, alongside the diverse types of nanoburrs that are currently under development and the various materials they’re crafted from. Understanding these intricacies is essential for appreciating the profound potential of this novel approach to arterial repair.
Introduction to Nanoburr Technology
Nanoburr technology represents a promising frontier in regenerative medicine, offering a novel approach to tissue repair. These minuscule, engineered structures, much smaller than a human hair, are designed to adhere specifically to damaged arterial walls and initiate the healing process. Their targeted delivery and unique adhesive properties hold significant potential for treating a wide range of vascular diseases, from atherosclerosis to traumatic injuries.
The concept is based on the natural tendency of the body to repair itself, but with the precision and control offered by nanotechnology.The fundamental principle behind nanoburr technology relies on the controlled release of specialized adhesive molecules on the nanoburr surface. These molecules, strategically positioned, recognize and bind to damaged tissue with high specificity, minimizing interference with healthy tissue.
This targeted adhesion is critical for ensuring efficient tissue repair and minimizing unwanted side effects. The key lies in the precise design of the nanoburr’s structure and the choice of biocompatible materials.
Nanoburr Types for Tissue Repair
Various types of nanoburrs can be engineered, each with its unique properties and potential applications. The choice of material and structure directly influences the nanoburr’s ability to adhere to damaged tissue, its biocompatibility, and its longevity within the body.
Tiny nanoburrs sticking to damaged arteries and repairing tissue is fascinating, right? It’s like nature’s own microscopic repair kit. However, this reminds me of a recent story about a fired contractor who apparently “kissed off Fannie Mae with a logic bomb” – fired contractor kisses off Fannie Mae with logic bomb. It’s crazy how seemingly unrelated events can highlight the complexities of modern systems and solutions, even down to the nanoscale of these tiny burrs.
Still, I’m more interested in the intricate details of how these nanoburrs work. They seem like a powerful tool for medical advancement.
Nanoburr Material Properties
The selection of materials for nanoburrs is crucial for their functionality and biocompatibility. The materials must be biocompatible, meaning they do not elicit an adverse immune response or cause inflammation in the body. Furthermore, the material should be strong enough to withstand the mechanical stresses within the vascular system, yet also degrade in a controlled manner to allow for natural tissue regeneration.
Nanoburr Type | Material | Adhesive Mechanism | Expected Longevity | Potential Applications |
---|---|---|---|---|
Collagen-based Nanoburrs | Collagen, chitosan | Specific binding to damaged collagen fibers in arteries | Several weeks | Treating vascular injuries, promoting endothelial regeneration |
Chitosan-based Nanoburrs | Chitosan, hyaluronic acid | Adhesion via electrostatic interactions and hydrogen bonding | Several months | Repairing injured blood vessels, promoting angiogenesis |
Bioglass Nanoburrs | Bioglass | Integration with bone tissue matrix, stimulating calcium phosphate deposition | Months to years | Repairing bone defects, promoting osseointegration |
Nanoburrs with bioactive peptides | Biocompatible polymers, bioactive peptides | Targeted binding to specific cell receptors | Weeks to months | Stimulating cell growth, promoting tissue regeneration |
Mechanisms of Tissue Repair: Tiny Nanoburrs Stick To Damaged Arteries And Repair Tissue
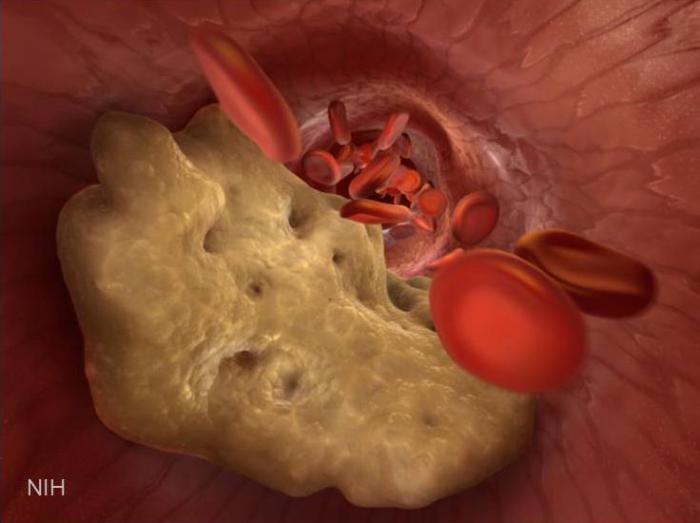
Nanoburrs, tiny structures designed to adhere to damaged arteries, offer a novel approach to tissue repair. Their precise targeting and unique interaction with biological processes promise significant advancements over traditional methods. Understanding the mechanisms behind both the damage and the repair is crucial to evaluating the potential of this technology.The process of arterial damage and repair is a complex interplay of cellular responses.
Damage, whether from injury or disease, initiates a cascade of events. Inflammation, characterized by the recruitment of immune cells, is an initial response. Subsequently, the body attempts to seal the damage through the formation of a blood clot, followed by the crucial process of tissue regeneration. This involves the proliferation of specialized cells like endothelial cells, smooth muscle cells, and fibroblasts, which are responsible for creating new tissue.
Disruptions in any of these steps can lead to long-term complications, such as atherosclerosis.
Biological Processes in Arterial Damage and Repair
Arterial damage triggers a series of biological responses. Inflammation, a protective mechanism, is initiated to contain the damage. Macrophages, a type of immune cell, engulf cellular debris and initiate the subsequent stages of repair. The formation of a platelet plug and the coagulation cascade help to stop bleeding. Following this, the body attempts to restore the damaged arterial wall.
Tiny nanoburrs sticking to damaged arteries and repairing tissue is pretty cool, right? Imagine the possibilities for treating cardiovascular disease. But, honestly, how much would you pay for that kind of groundbreaking medical news story to be widely disseminated? This article explores the value of online news dissemination and the impact it can have on public understanding of science.
Still, the potential for these tiny nanoburrs to revolutionize medicine is truly exciting.
Endothelial cells proliferate to line the inner surface of the vessel, while smooth muscle cells and fibroblasts lay down new connective tissue. This intricate process is essential for maintaining vascular integrity.
Nanoburr Interaction with Damaged Arterial Wall
Nanoburrs, with their precise targeting mechanism, adhere to the damaged arterial wall. Their unique design facilitates direct interaction with the damaged tissue, potentially accelerating the healing process. The controlled release of growth factors and biocompatible materials integrated into the nanoburrs can stimulate cell proliferation and differentiation. This targeted approach avoids the generalized effects of some traditional treatments.
The nanoburrs act as tiny scaffolding, providing structural support for the healing tissue.
Effectiveness Comparison with Traditional Methods
Traditional methods for arterial repair, such as stenting and bypass surgery, often involve significant invasiveness and potential complications. Nanoburr technology, by contrast, aims for a less invasive approach. This minimally invasive method may lead to reduced recovery times and lower risks of complications compared to traditional surgical interventions. Furthermore, the targeted delivery of growth factors through nanoburrs could lead to more effective repair and reduced long-term complications.
Tissue Repair Process Facilitation by Nanoburrs
Nanoburrs facilitate tissue repair through a multi-step process. First, the nanoburrs attach to the damaged area. Then, they release growth factors, stimulating the proliferation of endothelial cells and other necessary cells. The release of specific molecules could further promote the deposition of extracellular matrix components, strengthening the repaired tissue. The presence of the nanoburrs could also provide structural support to the developing tissue, enhancing the strength and integrity of the repair.
This process potentially accelerates the overall healing time.
Potential Long-Term Effects of Nanoburr Implantation
Long-term effects of nanoburr implantation are currently under investigation. Initial studies suggest a positive impact on tissue regeneration, with reduced scarring and inflammation. However, long-term effects, such as potential immune responses or interactions with existing medical implants, require further investigation. Careful monitoring and follow-up studies are crucial to understanding the long-term safety and efficacy of this innovative technology.
The impact on existing conditions like atherosclerosis or other vascular diseases will be a key focus of future research.
Tiny nanoburrs sticking to damaged arteries and repairing tissue is fascinating, right? Imagine the possibilities! This new technology could revolutionize treatments, but it also relies on advancements like Bluetooth SIG’s brushed-up Version 3.0, which is boosting connectivity for various medical devices. This improved connectivity is crucial for the precise control and real-time feedback needed for nanoburr applications, ultimately making these repairs more effective.
Nanoburr Design and Fabrication
Nanoburrs, these microscopic marvels, are poised to revolutionize tissue repair. Their intricate design and precise fabrication methods are critical for ensuring their efficacy and biocompatibility. The ability to precisely control their shape, size, and material composition is key to maximizing their adhesion to damaged arteries and promoting effective tissue integration.The fabrication process of nanoburrs is multifaceted, demanding advanced techniques to create these tiny structures with exceptional precision.
Various approaches, each with unique advantages and limitations, are currently being explored and refined.
Fabrication Methods
Various nanofabrication techniques are employed to create nanoburrs. These include nanoimprint lithography, which uses a mold to create patterns on a substrate material, and focused ion beam milling, a powerful technique that allows for precise sculpting of materials at the nanoscale. Another method is electron beam lithography, which uses an electron beam to create intricate patterns on a photosensitive material.
Each method offers unique capabilities and limitations, affecting the resulting nanoburr’s shape, size, and surface characteristics. The choice of method often depends on the desired nanoburr characteristics and the scale of production.
Design Elements for Optimal Tissue Adhesion and Integration
Crucial design elements for optimal tissue adhesion and integration include:
- Surface Topography: Nanoburrs with precisely engineered surface textures are essential. Microscopic protrusions or patterns can enhance the mechanical interlocking with the surrounding tissue. This is critical for effective adhesion and reducing the risk of detachment.
- Material Selection: Biocompatible materials, such as polymers or bioceramics, are paramount. The material’s biodegradability, stiffness, and surface chemistry significantly influence the body’s response to the nanoburr. Bioactive components can be incorporated to further promote tissue regeneration.
- Size and Shape: The dimensions of the nanoburr are critical. Optimizing the size and shape ensures proper interaction with the damaged tissue and prevents potential adverse reactions. The geometry, like the tip angle and burr depth, can be tailored to improve tissue integration.
- Surface Chemistry: Functionalization of the nanoburr surface with specific biomolecules can enhance cell adhesion and promote tissue regeneration. For example, specific proteins can be adsorbed or chemically bound to the surface to guide cell growth and repair.
Comparison of Nanoburr Shapes
The table below compares different nanoburr shapes and their respective advantages and disadvantages.
Shape | Advantages | Disadvantages |
---|---|---|
Cone | Enhanced mechanical interlocking, potentially increased surface area for interaction | Potential for increased tissue damage during insertion |
Sphere | Reduced risk of tissue damage, potential for better biocompatibility | May not achieve the same level of mechanical interlocking as cone-shaped nanoburrs |
Cylindrical | Simple fabrication, potential for enhanced surface area | May not provide optimal tissue interaction |
Evaluation Criteria for Nanoburr Efficacy
The efficacy of different nanoburr designs can be evaluated based on several criteria, including:
- In Vitro Cell Adhesion and Proliferation: Experiments assessing the ability of cells to adhere to and proliferate on the nanoburr surface are crucial.
- In Vivo Tissue Integration and Repair: Animal models provide a valuable platform for evaluating the nanoburr’s performance in promoting tissue regeneration within a living organism.
- Mechanical Strength and Stability: Assessing the mechanical properties of the nanoburr-tissue interface is essential to ensure long-term stability and prevent detachment.
- Biocompatibility and Toxicity: The material’s biocompatibility and potential toxicity must be thoroughly evaluated to prevent adverse reactions.
Optimizing Nanoburr Design for Arterial Damage
Optimizing nanoburr design for different types of arterial damage requires careful consideration of the specific characteristics of each damage type. For example, in cases of severe atherosclerosis, nanoburrs with larger burrs or increased surface area might be needed to facilitate adequate repair. For minor damage, smaller, more precisely shaped nanoburrs could be used to minimize potential damage to healthy tissue.
Understanding the specific damage mechanisms and the type of tissue affected will allow for tailoring nanoburr designs for optimal repair outcomes.
Clinical Applications and Potential Benefits
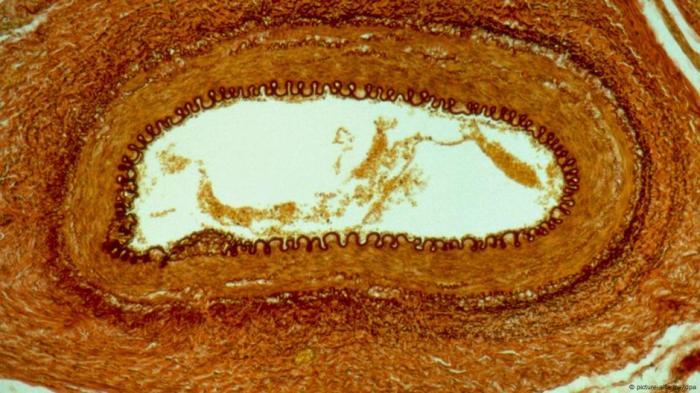
Nanoburr technology presents a compelling approach to cardiovascular repair, potentially revolutionizing the treatment of damaged arteries. This innovative method offers a targeted and precise approach, contrasting sharply with traditional therapies, which often suffer from limitations in terms of efficacy and side effects. The ability of nanoburrs to precisely adhere and stimulate tissue regeneration holds immense promise for treating a range of cardiovascular diseases.
Potential Clinical Applications, Tiny nanoburrs stick to damaged arteries and repair tissue
Nanoburrs can be strategically deployed to address various arterial pathologies. For instance, in cases of atherosclerosis, where plaque buildup narrows arteries, nanoburrs could be used to physically remove or stabilize the plaque. In instances of arterial injury due to trauma or surgery, nanoburrs could facilitate rapid and controlled tissue regeneration, minimizing the risk of complications and improving long-term functionality.
Potential Benefits Compared to Existing Therapies
Existing treatments for cardiovascular diseases often have limitations. Traditional methods, such as angioplasty and stenting, can sometimes lead to restenosis (re-narrowing) or other complications. Nanoburrs, on the other hand, offer a more targeted approach, potentially reducing the risk of these complications. Their ability to promote natural tissue regeneration could lead to improved long-term outcomes and reduced reliance on invasive procedures.
Applications in Treating Various Cardiovascular Diseases
Nanoburr technology shows promise in treating a range of cardiovascular diseases. In coronary artery disease, nanoburrs could be used to repair damaged vessels, restoring blood flow and mitigating the risk of heart attacks. In peripheral artery disease, they could stimulate the growth of new blood vessels, improving circulation in affected limbs. Moreover, nanoburrs could play a crucial role in treating aneurysms by strengthening weakened arterial walls.
Specific Scenarios of Nanoburr Utilization
Imagine a patient with a severely damaged artery after a traumatic injury. Traditional treatments might only provide temporary relief. Nanoburrs, however, could be precisely positioned to stimulate the growth of new tissue, leading to complete regeneration and long-term functional recovery. In another scenario, a patient with atherosclerosis could benefit from nanoburrs targeted at dissolving plaque, opening up the artery and restoring normal blood flow without the need for invasive procedures like angioplasty.
Comparison of Nanoburr Technology with Existing Methods
Feature | Nanoburr Technology | Existing Methods (e.g., Angioplasty/Stenting) |
---|---|---|
Mechanism of Action | Stimulates natural tissue regeneration, targeted delivery | Mechanical dilation, implantation of foreign devices |
Target Specificity | High, allowing for precise repair | Lower, potentially leading to complications |
Long-term Outcomes | Potentially superior, promoting natural healing | Variable, often requiring follow-up procedures |
Risk of Complications | Potentially lower, due to targeted delivery | Higher, associated with procedures like restenosis |
Cost | Potentially higher initially, but could lead to lower long-term costs | Potentially lower initially, but higher long-term costs due to complications |
Nanoburr technology holds the potential to revolutionize cardiovascular repair by offering a more targeted and effective approach to tissue regeneration.
Safety and Toxicity Considerations
The promise of nanoburrs for tissue repair hinges on their safe integration into the human body. While the potential benefits are substantial, careful consideration of potential risks and toxicity is paramount. This section explores the crucial safety aspects, emphasizing the importance of rigorous testing and thorough evaluation before clinical trials.
Potential Risks and Safety Concerns
Nanoburr implantation, like any medical procedure, carries inherent risks. Potential complications include allergic reactions, inflammatory responses, and immune system activation. Furthermore, the potential for nanoburrs to migrate to unintended locations or interact with other biological systems needs thorough investigation. The unique characteristics of nanomaterials, such as their size and surface properties, can influence their interaction with biological tissues, raising concerns about unintended consequences.
Methods for Assessing Biocompatibility
Assessing the biocompatibility of nanoburrs is crucial. Various in vitro and in vivo methods are employed to evaluate their interaction with biological systems. In vitro studies, such as cell culture assays, can assess the cytotoxicity of nanoburrs on different cell types. In vivo models, using animal studies, provide a more realistic assessment of their biodistribution, potential for inflammation, and long-term effects.
These studies are designed to mimic the human biological environment as closely as possible to predict potential reactions in humans.
Potential Long-Term Effects
Long-term effects of nanoburr implantation are a critical concern. Studies must investigate potential accumulation of nanoburrs in tissues over time, their impact on organ function, and any potential for chronic inflammation or tissue damage. The long-term effects are essential to understand, as nanoburrs are intended for long-term tissue integration. Similar to the use of synthetic materials in implants, the long-term behavior of nanoburrs needs meticulous study.
Methods for Evaluating the Safety Profile
A comprehensive safety profile for nanoburrs requires a multi-faceted approach. This involves a tiered system of testing, beginning with in vitro studies to evaluate material properties and cytotoxicity. Subsequent in vivo studies using animal models help assess biodistribution, inflammatory response, and potential long-term effects. The data collected from these studies is meticulously analyzed to identify potential safety concerns and guide the development of mitigation strategies.
Finally, clinical trials are necessary to confirm the safety profile in humans and refine the dosage and application methods.
Summary Table of Potential Risks and Mitigation Strategies
Potential Risk | Mitigation Strategy |
---|---|
Allergic reactions | Rigorous material characterization and pre-clinical studies to identify potential allergens. Formulation optimization to reduce immunogenicity. |
Inflammation | Surface modifications to minimize interactions with immune cells. Optimization of nanoburr design for reduced inflammatory response. |
Migration to unintended locations | Precise targeting mechanisms for nanoburrs. Careful consideration of material properties to reduce potential for migration. |
Toxicity | Comprehensive in vitro and in vivo toxicity studies. Selection of biocompatible materials and surface coatings. |
Long-term effects | Long-term animal studies to assess accumulation and potential chronic effects. Detailed monitoring in clinical trials. |
Future Directions and Research
Nanoburr technology for tissue repair is a rapidly evolving field, promising significant advancements in regenerative medicine. Current research is focused on optimizing nanoburr design, improving their biocompatibility, and exploring their potential beyond arterial repair. The potential for these tiny tools to revolutionize various medical fields is substantial, but rigorous research is essential to realize their full promise.
Current State of Research
Research into nanoburr technology for tissue repair is currently exploring a range of factors. This includes the development of more sophisticated fabrication techniques, the investigation of diverse materials for nanoburr construction, and the evaluation of various biocompatible coatings to enhance their interaction with the biological environment. Early clinical trials are underway to assess the safety and efficacy of nanoburrs in animal models, paving the way for future human trials.
Areas for Further Research
To enhance the effectiveness and safety of nanoburrs, further research is crucial. Optimizing the precise control over nanoburr deployment and interaction with the target tissue is vital. Further research into the mechanisms by which nanoburrs stimulate tissue regeneration is also required. Improving the long-term biocompatibility of nanoburrs and understanding their potential for causing inflammation or immune responses is essential.
Finally, the development of standardized protocols for nanoburr fabrication and characterization is critical for reproducibility and quality control.
Potential Applications in Other Medical Fields
The potential for nanoburrs extends beyond arterial repair. Researchers are investigating their use in bone regeneration, cartilage repair, and even the treatment of certain types of neurological disorders. The ability of nanoburrs to precisely target and stimulate specific tissues holds great promise for a wide range of medical applications. For example, in bone regeneration, nanoburrs could be designed to deliver growth factors directly to the fracture site, accelerating the healing process.
Potential Future Developments and Implications
Future developments in nanoburr technology may involve the integration of advanced imaging techniques for real-time monitoring of nanoburr deployment and tissue response. The development of smart nanoburrs that can respond to specific biochemical signals within the body could lead to more personalized and effective therapies. For example, nanoburrs that release drugs in a controlled manner could be used to treat tumors or infections.
These developments could lead to more targeted and effective therapies, significantly impacting the future of medicine.
Key Research Questions and Potential Solutions
Research Question | Potential Solution |
---|---|
How can we precisely control the deployment of nanoburrs within the target tissue? | Employing advanced imaging techniques, such as confocal microscopy or ultrasound, to guide nanoburr delivery in real-time. |
What are the long-term effects of nanoburrs on the surrounding tissue? | Conducting extensive in vivo studies in animal models to assess the long-term biocompatibility and potential inflammatory responses. |
How can we improve the biocompatibility of nanoburrs? | Surface modification of nanoburrs with biocompatible polymers or coatings, such as polyethylene glycol. |
Can nanoburrs be used for targeted drug delivery? | Designing nanoburrs with drug-carrying capabilities, allowing for localized drug release at the target site. |
Epilogue
In conclusion, tiny nanoburrs present a compelling avenue for repairing damaged arteries, potentially revolutionizing cardiovascular care. This innovative approach, rooted in nanotechnology, offers a promising alternative to traditional methods. While challenges remain in terms of safety and long-term effects, the potential benefits of nanoburr technology are significant, promising to improve patient outcomes in treating various cardiovascular diseases. Further research and development are crucial to fully realize the potential of this transformative technology.