Large Hadron Collider Gets Smashing Unveiling the Universe
Large hadron collider gets smashing! This incredible machine, a marvel of engineering and science, is smashing particles together at unprecedented speeds. We’ll delve into the historical context, the physics behind the collisions, potential implications, and the public perception surrounding this groundbreaking research. From the early days of particle accelerators to the complex detectors analyzing the results, this journey promises to unveil the secrets of the universe.
The Large Hadron Collider (LHC) at CERN is pushing the boundaries of our understanding of the universe. By accelerating particles to near-light speeds and colliding them, scientists hope to uncover new particles, forces, and insights into the fundamental building blocks of matter. This process, though complex, is at the heart of numerous groundbreaking discoveries.
Historical Context of the LHC
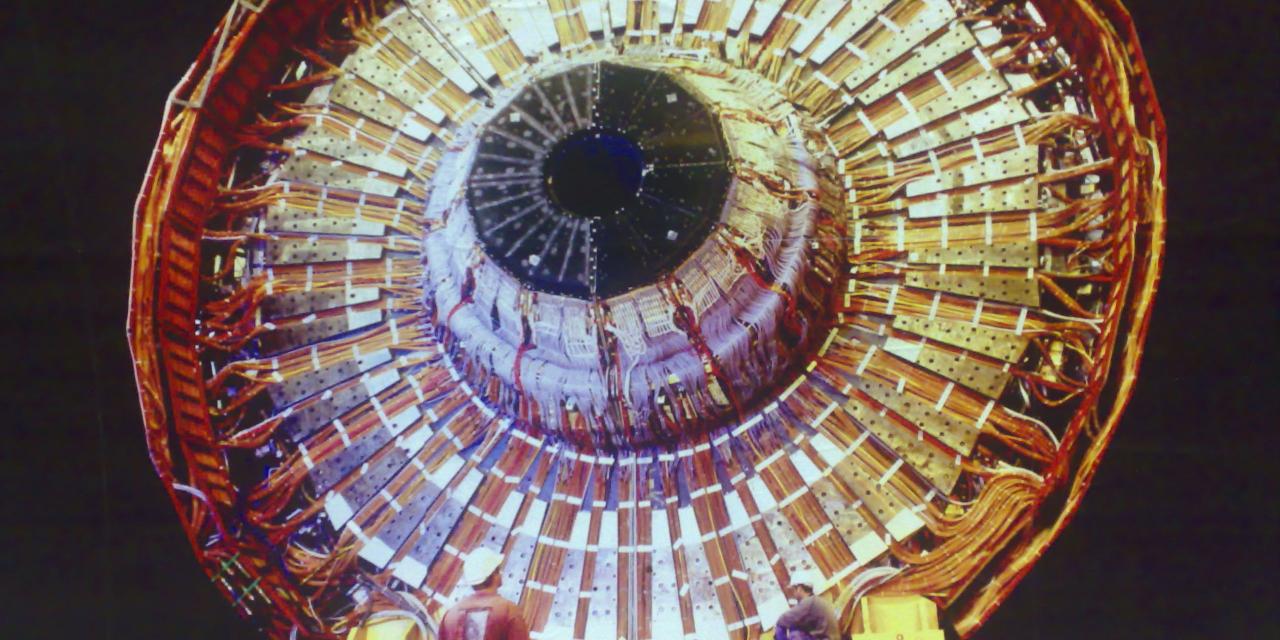
The Large Hadron Collider (LHC) stands as a testament to human ingenuity and a pivotal achievement in the pursuit of understanding the universe’s fundamental building blocks. Its construction, operation, and discoveries have deeply impacted our knowledge of particle physics, revealing secrets hidden within the subatomic realm. This exploration delves into the historical context, highlighting the journey from early particle accelerators to the groundbreaking research conducted at the LHC.The quest to unravel the secrets of matter has been a driving force in scientific advancement for decades.
Early particle accelerators, like the cyclotron and synchrotron, paved the way for the LHC. These machines, gradually increasing in size and energy, allowed scientists to probe deeper into the structure of atoms and discover new particles. This progression culminated in the LHC’s ability to explore energies previously inaccessible, opening new avenues for research.
Early Particle Accelerators and Milestones
Early experiments in particle physics involved the use of natural radioactive sources and early, rudimentary particle accelerators. The development of the cyclotron in the 1930s, which used a magnetic field to accelerate charged particles, marked a significant leap forward. This was followed by the synchrotron, which accelerated particles in a circular path using a rapidly varying magnetic field, allowing for higher energies.
These advancements progressively increased the energy and precision of experiments, revealing the existence of fundamental particles and the forces governing their interactions. Key milestones include the discovery of the positron, the pion, and the muon. These discoveries, made possible by these accelerators, fueled further research and the quest for more powerful tools to delve deeper into the subatomic world.
The Large Hadron Collider is getting smashing results, pushing the boundaries of particle physics. Meanwhile, significant changes are happening in the digital world, with ICANN, the organization that manages internet domain names, leaving the nest to explore new horizons. This independent move is fascinating, and hopefully, it will have a positive impact on the future of the internet.
It’s all a bit mind-blowing, really, and the LHC continues to impress with its groundbreaking work.
Scientific Motivations Behind the LHC
The primary motivation behind the LHC’s construction was to investigate the fundamental forces and particles governing the universe. Scientists sought to test the Standard Model of particle physics, a theoretical framework describing fundamental forces and particles. Further, the quest to discover the Higgs boson, a particle postulated to give mass to other particles, was a key driver. The model predicted the existence of the Higgs boson, but its detection remained elusive.
The LHC’s immense energy provided the necessary conditions to potentially confirm or refute these predictions.
Significant Discoveries by Preceding Accelerators
Particle accelerators played a crucial role in unveiling numerous fundamental particles and interactions. The discovery of the antiproton and anti-neutron, the pion, and the muon were all pivotal findings enabled by accelerators preceding the LHC. These discoveries expanded our understanding of the universe’s fundamental building blocks and the forces governing them.
The Large Hadron Collider is getting smashing results, pushing the boundaries of particle physics. While we marvel at these scientific feats, it’s worth remembering that the everyday hazards of a home office, as highlighted in a recent study, can be equally impactful, potentially leading to workplace injuries. For example, a computer accident study highlights hazards of home office emphasizes the importance of ergonomic setups.
Ultimately, whether it’s the intricate workings of the universe or the simple act of using a computer, safety and awareness are key elements in ensuring smooth operations and avoiding costly mishaps, just as the LHC continues its groundbreaking work.
International Collaboration in the LHC Project
The LHC project exemplifies international collaboration. Thousands of scientists and engineers from numerous countries contributed to its design, construction, and operation. This international collaboration was crucial for the project’s success, drawing on the expertise and resources of various nations. The sharing of knowledge, resources, and manpower allowed the project to surpass national limitations.
Timeline of Key Events in LHC Operations
- 2008: The LHC’s first beams were successfully circulated, marking a significant milestone in its operational history.
- 2012: The discovery of the Higgs boson, a particle predicted by the Standard Model, validated a crucial aspect of our understanding of particle physics.
- 2015: The LHC continued to operate, gathering extensive data on particle interactions.
- 2018: The LHC underwent a significant upgrade, enhancing its energy and capabilities.
Comparison of LHC to Previous Accelerators
Accelerator | Year | Energy (TeV) | Key Discoveries |
---|---|---|---|
CERN Proton Synchrotron (PS) | 1959 | 28 | Early particle discoveries |
CERN Super Proton Synchrotron (SPS) | 1976 | 400 | Further particle discoveries and understanding of interactions |
Large Hadron Collider (LHC) | 2008 | 14 | Higgs boson, precise measurements of other particles and forces |
The “Smashing” Phenomenon
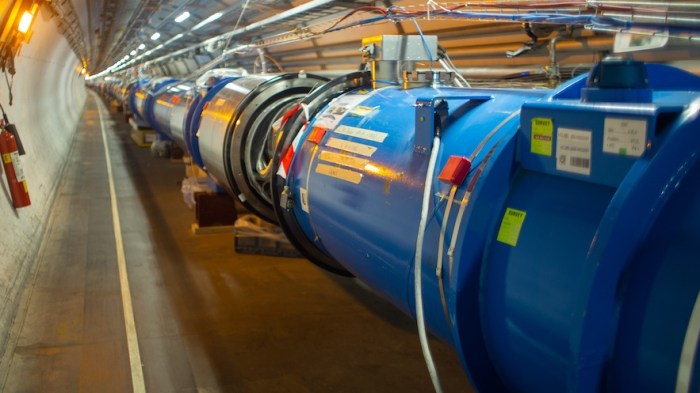
The Large Hadron Collider (LHC) achieves its remarkable results through the controlled “smashing” of particles at incredibly high speeds. This process, akin to a microscopic collision of titans, generates a flurry of new particles and reveals fundamental forces in the universe. Understanding these collisions and their aftermath is key to unlocking the secrets of the cosmos.The physics behind these collisions lies in Einstein’s famous equation, E=mc².
When particles collide with enough energy, the energy is converted into mass, creating new particles. This process is not just theoretical; it is a fundamental principle governing the universe, from the Big Bang to the workings of stars.
Particle Types in Collisions
Particle collisions at the LHC involve a wide range of fundamental particles, primarily protons. These protons, comprising quarks and gluons, are accelerated to near-light speeds before colliding. Other particles, like electrons and positrons, are also used in some experiments to study different aspects of particle interactions. The collision of these high-energy particles triggers a cascade of reactions, generating a multitude of secondary particles.
The study of these particles and their interactions is crucial to understanding the fundamental forces of nature.
Detection and Analysis Methods, Large hadron collider gets smashing
Detecting and analyzing the products of these collisions is a complex undertaking. Sophisticated detectors, placed strategically around the collision points, record the trajectories, energies, and types of particles produced. These detectors are meticulously designed to withstand the extreme conditions and record the vast amounts of data generated during each collision. The data is then subjected to complex algorithms to identify the various particles and their interactions.
This data analysis is crucial for identifying rare events and drawing conclusions about the fundamental laws of physics.
Equipment for Collision Monitoring and Analysis
The LHC experiments utilize sophisticated equipment for monitoring and analyzing collisions. This includes powerful computers to process the enormous data streams, sophisticated algorithms to identify patterns in the data, and highly sensitive detectors that capture the details of the interactions. The accuracy of the data analysis depends significantly on the quality and precision of the equipment used.
Data Analysis Techniques
Data analysis techniques play a vital role in interpreting the results of these collisions. Statistical methods, machine learning algorithms, and pattern recognition techniques are employed to sift through the vast amount of data and identify significant results. The goal is to extract meaningful information from the complex data generated, allowing scientists to draw conclusions about the fundamental laws of physics.
For instance, identifying a specific particle type or interaction pattern requires advanced statistical tools to distinguish it from background noise.
Experimental Setup for Collision Studies
The experimental setup for studying particle collisions at the LHC involves several key components. These include the accelerating structures, the collision point itself, and a complex array of detectors placed strategically around the collision point. Each detector has a specific function in capturing and analyzing the particles produced during the collision. This complex system of interconnected detectors is crucial for identifying the properties of the newly generated particles.
The precise positioning and design of each detector is critical to capturing the necessary information about the collisions.
Particle Detector Types
- Silicon Tracking Detectors: These detectors use silicon sensors to track the paths of charged particles, measuring their momentum and charge. This information is vital for reconstructing the events of the collision.
- Electromagnetic Calorimeters: These detectors measure the energy of electrons and photons produced in the collisions. They employ sophisticated materials to absorb and measure the energy of these particles.
- Hadronic Calorimeters: These detectors measure the energy of hadrons, which are composite particles containing quarks. Different materials are used to absorb the energy of hadrons, and the signals are recorded to determine their energies.
- Muon Detectors: Muons are a type of lepton that can travel long distances and are detected by specialized detectors using magnetic fields to measure their momentum.
Detector Type | Function | Key Measurements |
---|---|---|
Silicon Tracking Detectors | Track charged particles | Momentum, charge, trajectory |
Electromagnetic Calorimeters | Measure energy of electrons and photons | Energy, direction |
Hadronic Calorimeters | Measure energy of hadrons | Energy, direction |
Muon Detectors | Detect muons | Momentum, direction |
Potential Implications and Discoveries
The Large Hadron Collider (LHC) isn’t just about smashing particles; it’s about unraveling the very fabric of the universe. Potential discoveries could revolutionize our understanding of fundamental forces, matter, and the cosmos itself. The collisions at unprecedented energies could reveal new particles, shed light on dark matter and dark energy, and potentially unveil previously unknown phenomena. These discoveries, though theoretical, could have profound implications for various fields of science and technology.The expected outcomes of these high-energy collisions are multifaceted.
Previous experiments, such as those at the Stanford Linear Accelerator Center (SLAC) and the Tevatron, have yielded valuable insights into particle physics. However, the LHC’s unparalleled energy allows for probing energies and scales not previously accessible. This has the potential to reveal particles or interactions that eluded detection in the past, leading to breakthroughs in our understanding of fundamental physics.
Potential New Particles and Phenomena
The collisions at the LHC could potentially produce new particles beyond the Standard Model of particle physics. These hypothetical particles, if discovered, could dramatically alter our current understanding of the universe. While the Standard Model has been remarkably successful in describing known particles and their interactions, it leaves many unanswered questions, such as the nature of dark matter and the unification of forces.
The LHC’s exploration of the unknown could provide crucial answers.
Comparison with Previous Experimental Results
Previous experiments, particularly those at the Tevatron and LEP, have already provided valuable data about particle interactions at high energies. The LHC surpasses these predecessors in terms of energy and precision, allowing for a more thorough exploration of the energy landscape. The data collected by the LHC will be compared to and contrasted with previous results to identify discrepancies or new phenomena.
This comparison is crucial for verifying the reliability and validity of the findings.
Breakthroughs in Understanding Fundamental Physics
Potential breakthroughs in fundamental physics could emerge from the LHC’s explorations. These breakthroughs could lead to a more complete understanding of the universe’s fundamental forces, particles, and interactions. For instance, a discovery of new particles or interactions could provide evidence for supersymmetry, string theory, or other theoretical frameworks.
Significance for Our Understanding of the Universe
The implications of potential discoveries at the LHC extend far beyond particle physics. A deeper understanding of the universe’s fundamental constituents could reveal the nature of dark matter and dark energy, two enigmatic components that make up a significant portion of the cosmos. These discoveries could also offer new insights into the early universe and the conditions that led to its formation.
Applications in Science and Technology
The findings from the LHC could have applications in various fields of science and technology. For example, new materials with unique properties could be designed based on the insights gained from the fundamental interactions studied at the LHC. Advances in computing, energy storage, and medical imaging could also arise from the development of new technologies inspired by the discoveries at the LHC.
The Large Hadron Collider is getting some serious smashing action, pushing the boundaries of particle physics. But while these subatomic particles are colliding, it’s worth reflecting on the complexities of economic stimulus plans, like the tale of 2 stim plans US sold out, where Intel seemingly got it right here. Ultimately, these groundbreaking discoveries, whether in the quantum realm or the realm of economics, continue to shape our understanding of the universe, from the smallest particles to the largest market forces.
So, back to the smashing at the LHC!
Table of Potential New Particles or Phenomena
Particle Name | Properties | Interactions |
---|---|---|
Supersymmetric Partners | Hypothetical particles with similar properties to known particles but differing spins. | Interactions with known particles, potentially shedding light on the unification of forces. |
Dark Matter Candidates | Particles that interact very weakly with ordinary matter. | Interactions could be extremely weak, explaining the lack of observation in previous experiments. |
Extra Dimensions | Hypothetical spatial dimensions beyond the three we perceive. | Interactions could be manifested as unusual effects at high energies, potentially providing clues about the structure of space-time. |
Public Perception and Impact: Large Hadron Collider Gets Smashing
The Large Hadron Collider (LHC) represents a fascinating blend of scientific ambition and public intrigue. Its sheer scale and the potential for groundbreaking discoveries have captivated the world, while also sparking considerable discussion about the ethics and societal implications of such large-scale projects. This section delves into the public’s engagement with the LHC, the role of media in shaping perceptions, and the importance of transparent communication about scientific endeavors.
Public Interest and Understanding
The LHC has garnered significant public interest, fueled by its potential to unveil fundamental truths about the universe. This interest, however, is often accompanied by a degree of scientific illiteracy, making clear communication of the research crucial. While many appreciate the potential benefits, some remain skeptical, often due to a lack of understanding of the complex scientific processes involved.
Educational initiatives and accessible explanations are therefore vital to bridging the gap between scientific discovery and public comprehension. The potential for public engagement, particularly among younger generations, is significant and should be leveraged.
Media’s Role in Shaping Perception
The media plays a pivotal role in shaping public understanding of the LHC. News stories, documentaries, and popular science articles can either clarify or distort the complexities of the research. Effective communication, employing accessible language and avoiding sensationalism, is essential. Conversely, inaccurate or overly simplified portrayals can lead to misconceptions and potentially undermine public trust in science.
Responsible reporting that highlights both the potential benefits and risks is vital. The media, as a powerful tool, can either foster informed dialogue or perpetuate misunderstandings.
Communicating Scientific Discoveries to the Public
Effective communication of scientific discoveries to the public is paramount. This requires clear and concise explanations that avoid jargon and focus on the significance of the research. Scientists and communicators must actively engage with the public, utilizing various channels like public lectures, social media, and interactive exhibits. Visual aids and analogies can further enhance comprehension and facilitate the dissemination of complex information in a readily understandable format.
This process helps foster a culture of scientific literacy.
Ethical Considerations of Large-Scale Scientific Projects
Large-scale scientific projects like the LHC raise important ethical considerations. These include the potential for unintended consequences, the equitable distribution of benefits and risks, and the responsibility of scientists to address potential harms. Open dialogue and transparent decision-making processes are essential. Stakeholder involvement in the project’s design, execution, and impact assessment is vital. These projects necessitate ethical frameworks to guide their development and deployment.
Societal Implications of the Research
The LHC’s research has potential benefits and risks for society. The potential for advancements in fundamental physics could lead to technological breakthroughs, impacting various sectors, such as medicine, energy, and materials science. Conversely, concerns exist about the potential for misuse of such discoveries. Responsible research and development, coupled with ethical considerations, are crucial to mitigating potential risks.
A nuanced approach that considers both potential benefits and hazards is essential.
Public Communication Methods
Method | Target Audience | Effectiveness |
---|---|---|
Public lectures and seminars | General public, students, and enthusiasts | High potential for engagement and understanding; facilitates direct interaction. |
Educational outreach programs | School-aged children and youth | Fosters early interest and understanding; promotes long-term engagement with science. |
Interactive exhibits and museums | General public, families, and tourists | Engaging and interactive approach; increases accessibility and understanding of complex topics. |
Social media and online platforms | Broad audience, including social media users and internet enthusiasts | High reach and potential for quick dissemination of information; requires careful management to maintain accuracy. |
Documentary films and popular science articles | Broad audience interested in science and technology | Captivating and engaging approach to reaching a broad spectrum of viewers; can be impactful in sparking interest. |
Technical Aspects of the Collisions
The Large Hadron Collider (LHC) isn’t just about smashing particles; it’s about mastering incredibly complex engineering to achieve those collisions. This intricate dance of physics and technology requires a precise choreography of acceleration, confinement, and detection. The engineering challenges are immense, pushing the boundaries of what’s possible in particle physics.The LHC’s primary goal is to create high-energy collisions between particles, which in turn allows scientists to observe the fundamental forces of nature and the particles that make up the universe.
Achieving these collisions requires a series of meticulously designed systems, from the powerful magnets that steer and accelerate particles to the sophisticated detectors that record the aftermath of the collisions.
Accelerating Particles to Extreme Speeds
Particle acceleration is a crucial aspect of the LHC. The process involves propelling protons to extremely high speeds using a series of accelerating structures. These structures, called cavities, use radio frequency (RF) waves to boost the energy of the protons. Protons are accelerated in stages, with each stage increasing their energy until they reach the desired collision energy.
The intricate design of these accelerating cavities is critical for maintaining the beam’s stability and integrity.
The Role of Superconductivity
Superconductivity plays a vital role in the LHC’s ability to achieve and maintain the high magnetic fields required to steer and focus the beams. Superconducting magnets, cooled to extremely low temperatures, allow for the generation of strong magnetic fields with minimal energy loss. This efficiency is crucial for the LHC’s overall energy consumption and operational cost. Superconducting materials exhibit zero electrical resistance below a critical temperature, allowing for efficient energy transfer and enabling the creation of powerful magnetic fields with a smaller power source.
The Complexity of the LHC Infrastructure
The LHC’s infrastructure is incredibly complex, encompassing several crucial systems that must work in perfect harmony. The vacuum systems are critical to maintain a near-perfect vacuum within the accelerator’s tubes, minimizing collisions between the particles and the residual gas molecules. This near-vacuum environment is essential for allowing the protons to travel at high speeds without significant energy loss.
The cryogenic systems are equally important, as they cool the superconducting magnets to extremely low temperatures, enabling superconductivity. This delicate balance of temperature and pressure ensures the stability and performance of the entire system.
Types of Magnets Used in the LHC
The LHC utilizes various types of magnets to control and guide the accelerated proton beams. Different types of magnets are needed to perform specific functions, such as bending the beams or focusing them.
Magnet Type | Function | Materials |
---|---|---|
Dipole Magnets | Bend the particle beam | Niobium-titanium (NbTi) or Niobium-tin (Nb3Sn) |
Quadrupole Magnets | Focus the particle beam | Niobium-titanium (NbTi) or Niobium-tin (Nb3Sn) |
Sextupole Magnets | Correct beam distortions | Niobium-titanium (NbTi) or Niobium-tin (Nb3Sn) |
The choice of materials for these magnets is crucial. Niobium-titanium (NbTi) is often used in the bending magnets due to its high field strength and relative cost-effectiveness, while niobium-tin (Nb3Sn) is employed in magnets requiring higher field strengths. The materials’ properties, including their critical temperature and field strength, directly influence the design and performance of the entire system.
Ending Remarks
In conclusion, the Large Hadron Collider’s smashing collisions are not just scientific experiments; they’re a testament to human ingenuity and a profound quest to understand the universe’s mysteries. From the intricate technical aspects to the potential societal implications, the LHC’s impact resonates far beyond the laboratory. This ongoing research promises to unlock new discoveries and reshape our understanding of the cosmos.