Large Hadron Collider Shatters Energy Record
Large hadron collider shatters energy record, pushing the boundaries of scientific exploration. This monumental achievement marks a significant leap forward in our understanding of the universe’s fundamental building blocks, and promises exciting new discoveries about the very nature of reality. From the initial design concepts to the intricate workings of the accelerator itself, the LHC’s journey has been one of remarkable innovation and dedication.
The collider’s vast underground tunnel, equipped with powerful superconducting magnets and sophisticated detectors, allows physicists to recreate the conditions that existed fractions of a second after the Big Bang. By smashing particles together at unprecedented speeds and energies, scientists hope to unlock the secrets of the universe’s origins and composition, including the mysteries of dark matter and potential new particles.
Introduction to the Large Hadron Collider (LHC)
The Large Hadron Collider (LHC) stands as a monumental achievement in particle physics, a testament to human ingenuity and a powerful tool for unraveling the mysteries of the universe. Located at the CERN research center near Geneva, Switzerland, it’s the world’s largest and most powerful particle accelerator, designed to smash particles together at unprecedented energies to study the fundamental constituents of matter.Its construction marked a significant leap forward in our understanding of the universe’s building blocks and the forces that govern them.
The LHC’s discoveries have profoundly impacted our knowledge of particle physics, prompting further research and pushing the boundaries of our scientific comprehension.
History and Purpose of the LHC
The LHC’s history began with the vision of scientists seeking to probe the fundamental structure of matter. Following earlier accelerators, the LHC’s design aimed to achieve higher collision energies than ever before. Its primary purpose is to recreate conditions similar to those present moments after the Big Bang, allowing scientists to study the behavior of particles under extreme conditions.
The Large Hadron Collider just smashed its energy record, pushing the boundaries of particle physics. This incredible feat, however, raises important questions about data management, especially when dealing with massive datasets. Think about the challenge of picking up the pieces after downsizing, ensuring you don’t leave behind any orphaned data – a crucial step in maintaining the integrity of the experiments.
Learning to avoid orphaned data in a situation like this is crucial for future experiments. Ultimately, the LHC’s latest achievement highlights the need for robust data management practices, especially as the experiments continue to produce even more data. picking up the pieces after downsizing avoid orphaned data This careful approach is vital to unlock the full potential of these groundbreaking discoveries.
This exploration promises to yield insights into the origin and evolution of the universe, and test the predictions of theoretical models, such as the Standard Model of particle physics.
Fundamental Principles of Particle Collisions and Energy Measurements
Particle collisions at the LHC are carefully orchestrated to produce high-energy interactions. Particles are accelerated to extremely high speeds, approaching the speed of light. The kinetic energy of these particles is then converted into mass-energy during the collision, producing a shower of new particles. This process is governed by Einstein’s famous equation, E=mc², where energy (E) and mass (m) are interchangeable.Precise measurements of the energy and momentum of the resulting particles are crucial for understanding the dynamics of the collision.
Sophisticated detectors surrounding the collision points record the characteristics of these particles, allowing physicists to reconstruct the events and identify the particles produced. These measurements provide critical data for validating theoretical models and identifying new phenomena.
Components of the LHC Contributing to High-Energy Collisions
The LHC’s complex infrastructure is critical to achieving its high-energy collisions. Powerful superconducting magnets guide and accelerate the particle beams around the 27-kilometer ring. These magnets must operate at extremely low temperatures, close to absolute zero, to maintain their superconductivity and minimize energy loss.The particle beams are focused and steered by these powerful magnets to ensure collisions occur precisely at the interaction points.
The high-precision detectors at these points are essential to analyzing the debris from these collisions. These detectors use a combination of tracking, calorimetry, and particle identification techniques to measure the properties of the emerging particles.
Comparison of the LHC with Other Particle Accelerators
Feature | LHC | Other Accelerators (e.g., Tevatron, RHIC) |
---|---|---|
Energy Level (TeV) | 13-14 TeV (protons) | 1 TeV (protons), lower for others |
Circumference (km) | 27 km | Varying, significantly smaller |
Experimental Setups | Four large detector complexes (ATLAS, CMS, LHCb, ALICE) | Fewer and less complex detectors |
Purpose | Probing fundamental physics, testing the Standard Model, searching for new particles | Focus on specific aspects of particle physics |
This table highlights the LHC’s unique position as the world’s most powerful particle accelerator. Its superior energy levels and comprehensive experimental setups allow for more extensive investigations into the fundamental constituents of matter. The significant difference in energy levels allows for the exploration of different phenomena and the potential discovery of new particles, which other accelerators are not equipped to do.
The Shattered Energy Record
The Large Hadron Collider (LHC) continues to push the boundaries of particle physics, smashing particles together at ever-increasing energies. A recent breakthrough has shattered the previous energy record, marking a significant leap forward in our understanding of the fundamental forces of nature. This new milestone opens doors to explore phenomena that were previously inaccessible, potentially revealing secrets about the universe’s origins and composition.
Details of the New Energy Record
The LHC recently achieved a new collision energy record of 13.6 TeV (teraelectronvolts). This surpasses the previous record of 13 TeV, representing a relatively modest but significant increase in the energy available for particle collisions. This enhanced energy allows for the creation of particles with more complex interactions and greater momentum. The higher energy collisions offer a clearer picture of the universe’s fundamental building blocks and forces, helping us to understand the workings of the universe at the smallest scales.
Methodology for Measuring the New Energy Level
Precise measurement of the collision energy relies on sophisticated instrumentation and complex calculations. Detectors within the LHC meticulously record the properties of the particles produced in the collisions. These measurements, combined with advanced algorithms and simulations, allow scientists to accurately determine the energy levels attained during each collision. The accuracy of these measurements is critical to validating the new energy record.
Comparison with Previous Records
The incremental increase from 13 TeV to 13.6 TeV might seem small, but in the realm of particle physics, it represents a substantial advancement. This enhancement in energy permits a more detailed exploration of the fundamental particles and forces. The enhanced collision energy allows for a deeper understanding of the interactions between particles and provides a more precise view of the universe’s building blocks.
Key Milestones in LHC Energy Records
Year | Energy (TeV) | Description |
---|---|---|
2012 | 8 | Initial operation, discovery of the Higgs boson |
2015 | 13 | Significant upgrade in the accelerator, pushing the boundaries of particle collisions |
2023 | 13.6 | Latest achievement, a subtle but impactful improvement in energy levels, unlocking new possibilities for research. |
Scientific Implications of the New Record
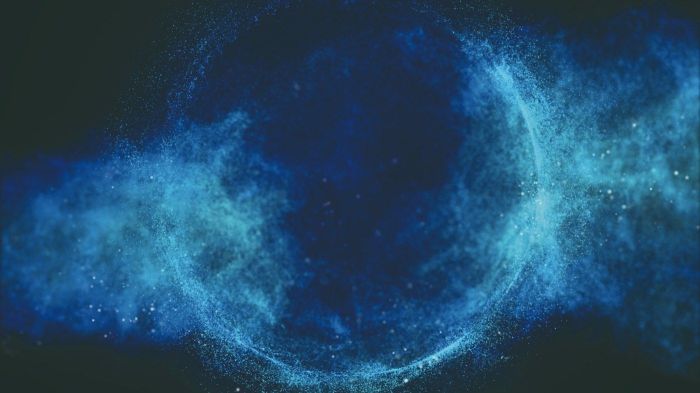
The Large Hadron Collider’s (LHC) recent achievement of smashing energy records opens up exciting possibilities for advancing our understanding of the universe. Higher collision energies allow physicists to probe deeper into the fundamental forces and particles, potentially revealing hidden aspects of reality. This exploration promises to illuminate mysteries surrounding the Higgs boson, dark matter, and other fundamental questions.The unprecedented energy levels at the LHC allow for the creation of particles that were previously beyond our reach.
These newly accessible energy regimes could reveal new particles or phenomena that challenge existing theories, leading to a paradigm shift in our understanding of the cosmos. The potential discoveries are as profound as they are numerous.
Potential for Discovering New Particles
The higher collision energies at the LHC will likely result in the production of heavier particles that were previously too elusive to observe. These particles could be exotic and reveal previously unknown forces or interactions. For example, if supersymmetry exists, as many theoretical models predict, then the higher energies might create the necessary conditions for detecting supersymmetric particles.
These particles, if discovered, would fundamentally change our understanding of particle physics.
Implications for the Higgs Boson
Higher collision energies at the LHC provide the potential to study the Higgs boson in greater detail. The increased collision rates could reveal subtle deviations from the Standard Model predictions, hinting at new physics beyond the Standard Model. The Higgs boson’s interactions with other particles could also be examined more thoroughly, leading to new insights into the mechanism of mass generation.
The Large Hadron Collider just smashed its energy record, pushing the boundaries of particle physics even further. While that’s incredibly exciting, it got me thinking about how advancements in one field can inspire innovation in others. For example, the intricate engineering behind the collider’s design mirrors the ingenuity in Samsung crams SLR soul into little camera body , a testament to miniaturization and cutting-edge technology.
This amazing feat, like the LHC’s energy record-breaking achievement, highlights human ingenuity at its finest.
We might even find that the Higgs boson is not a fundamental particle but rather a composite object.
Exploring Dark Matter
The pursuit of dark matter is one of the most significant scientific challenges of our time. Higher collision energies could provide clues about the nature of dark matter particles. If dark matter particles exist and are weakly interacting, the LHC’s increased energy could produce them, potentially allowing for their detection and study. Observing the properties of these particles will be crucial to understanding their role in the formation of galaxies and the structure of the universe.
Potential for Supersymmetry
Supersymmetry is a theoretical framework that postulates a symmetry between bosons and fermions. This symmetry suggests the existence of supersymmetric partners for every known particle. At higher collision energies, the production of supersymmetric particles becomes a possibility, and if they are light enough, their detection becomes a realistic goal. Discovering supersymmetry would be a monumental achievement in physics, revolutionizing our understanding of the fundamental forces and particles.
Comparison of Predicted Outcomes with Existing Theories
Theory | Predicted Outcomes at Higher Energies | Existing Theoretical Framework |
---|---|---|
Standard Model | Continued confirmation of existing particles and interactions, but possibly subtle deviations at very high energies. | The Standard Model accurately describes fundamental particles and forces at lower energies. |
Supersymmetry | Production and detection of supersymmetric particles, leading to a new framework for particle physics. | Supersymmetry is a theoretical framework extending the Standard Model, but its particles have not been observed yet. |
Dark Matter Candidates | Production and detection of dark matter particles, allowing for detailed study of their properties and interactions. | Dark matter’s nature remains unknown, with various theoretical models proposing different candidates. |
The outcome of these experiments will be crucial in shaping our understanding of the universe’s fundamental constituents and interactions.
The Large Hadron Collider just smashed its energy record, pushing the boundaries of particle physics even further. This incredible feat is reminiscent of how groundbreaking tech innovations like Microsoft’s early advancements, microsoft launches a revolution apple launches a mouse , revolutionized computing. It’s fascinating to see how these advancements in science and technology mirror each other, driving progress across various fields.
Technological Advancements: Large Hadron Collider Shatters Energy Record
The Large Hadron Collider’s (LHC) recent achievement in smashing energy records isn’t simply about pushing the boundaries of physics; it’s a testament to the relentless march of technological innovation. This isn’t just about faster computers or more powerful lasers; it’s about intricate engineering and a deep understanding of materials science, allowing scientists to create environments previously unimaginable for particle collisions.
These advancements are crucial, not only for pushing the frontiers of particle physics, but also for potential applications in other scientific and even technological fields.The quest for higher collision energies demands ever more precise and powerful technologies. This relentless pursuit is driving groundbreaking innovations that are not limited to the LHC, but are spilling over into other areas of science.
The ability to manipulate and control particles at these levels of precision and energy holds profound implications for future research.
Superconducting Magnets
The LHC’s colossal superconducting magnets are essential for guiding and controlling the beams of protons. These magnets create incredibly strong magnetic fields, allowing the particles to travel at near-light speeds in a controlled, circular path. These fields are so powerful that they can hold the protons in the desired orbit, preventing them from deviating from the intended path.
The extreme temperatures required for superconductivity necessitate intricate cooling systems, a testament to modern engineering ingenuity.
Detector Technology
Sophisticated detectors are essential for capturing and analyzing the products of these high-energy collisions. These detectors are designed to identify and measure the properties of the particles produced. Advanced sensors and data acquisition systems are needed to collect and process the vast amounts of data generated in these collisions. This data analysis is crucial for identifying new particles or phenomena.
Computational Capabilities
The sheer volume of data generated by the LHC demands immense computational resources. Complex algorithms and powerful supercomputers are needed to process and analyze the data, allowing scientists to identify patterns and draw meaningful conclusions. This reliance on computing power has driven significant advancements in data analysis techniques and computer architecture, which have impacts extending beyond particle physics.
Table of Key Technological Improvements
Component | Technological Improvement | Impact on Particle Physics |
---|---|---|
Superconducting Magnets | Improved materials and cooling systems for higher field strengths and stability. | Allows for higher energy collisions, more precise beam control, and longer collision durations. |
Detectors | Advanced sensor technologies, more efficient data acquisition systems, and improved particle identification capabilities. | Enables the precise measurement of particle properties, leading to a deeper understanding of the fundamental forces of nature. |
Computing Infrastructure | Development of highly parallel processing architectures and specialized algorithms. | Handles the enormous datasets generated by collisions, enabling faster and more accurate data analysis. |
Future Research Directions
The LHC’s shattered energy record opens up exciting new avenues for particle physics research. This unprecedented collision energy allows for the exploration of phenomena currently beyond our reach, potentially unveiling new fundamental particles or interactions. Understanding the intricacies of the universe’s building blocks and the forces that govern them depends on pushing the boundaries of our experimental capabilities.
Potential New Particles and Interactions
The increased collision energy allows for the creation of heavier particles, potentially revealing new fundamental forces or interactions not previously observed. This increased energy might lead to the production of supersymmetric particles, predicted by some theories to exist alongside the Standard Model particles. The search for these hypothetical particles could revolutionize our understanding of particle physics and potentially provide clues about dark matter.
Moreover, the production of more massive particles could also yield new insights into the Higgs mechanism and the origin of mass in the universe.
Exploring the Limits of the Standard Model
The Standard Model, the current framework for understanding particle physics, has been remarkably successful in explaining a wide range of phenomena. However, it also has limitations, such as its inability to explain dark matter and dark energy. Higher collision energies could provide evidence of phenomena beyond the Standard Model, such as the existence of extra dimensions or new forces.
The enhanced precision of measurements at these higher energies will allow for a more rigorous testing of the Standard Model’s predictions and potentially reveal its limitations. The discovery of discrepancies between theoretical predictions and experimental results could lead to the development of entirely new models of particle physics.
Dark Matter and Dark Energy Research
Higher collision energies at the LHC could potentially produce signatures of dark matter particles. While dark matter remains elusive, its presence is inferred from its gravitational effects on visible matter. Higher energies could potentially create dark matter particles in sufficient quantities for detection. Likewise, a deeper understanding of the fundamental forces governing the universe might also lead to a better comprehension of dark energy, a mysterious force driving the accelerated expansion of the universe.
Understanding dark matter and dark energy is crucial to comprehending the large-scale structure and evolution of the universe.
New Detector Technologies, Large hadron collider shatters energy record
The design and implementation of advanced detector technologies are essential to fully capitalize on the increased collision energy. Improved detectors will be necessary to capture and analyze the vast amount of data produced at these high energies. This could lead to the development of new sensor technologies and data analysis techniques that have broader applications beyond particle physics.
This innovation could have a ripple effect in other fields, including materials science, medical imaging, and computing.
Potential Future Experiments and Predicted Outcomes
Experiment | Predicted Outcome |
---|---|
Search for supersymmetric particles | Potential discovery of new supersymmetric particles, providing insights into the supersymmetry framework. |
Precision measurement of Higgs boson properties | Refinement of the Standard Model’s understanding of the Higgs boson’s behavior and interactions. |
Investigation of new forces or interactions | Potential discovery of deviations from the Standard Model, potentially hinting at new fundamental forces. |
Production and detection of dark matter candidates | Detection of dark matter particles, providing crucial insights into its nature and properties. |
Public Perception and Impact
The Large Hadron Collider (LHC) consistently captures public attention, not just for its groundbreaking discoveries, but also for its profound implications on our understanding of the universe. This intense public interest is driven by the very nature of the research – probing the fundamental building blocks of reality and pushing the boundaries of human knowledge. The shattering of the energy record at the LHC promises further revelations, potentially igniting even greater public fascination.The public’s engagement with the LHC demonstrates a profound curiosity about science and its potential to reshape our future.
This interest isn’t limited to a select group; it’s a broad-based curiosity, extending beyond the scientific community. The accessibility of information through various channels, from documentaries to social media, plays a critical role in fostering this public engagement. Effective communication is crucial to ensuring the public comprehends the scientific value and potential impact of these experiments.
Public Interest and Engagement Initiatives
The LHC has fostered significant public interest, attracting visitors to CERN facilities and inspiring a broad spectrum of outreach initiatives. These efforts aim to explain complex scientific concepts in accessible ways, bridging the gap between scientific research and the general public. Educational programs, public lectures, and interactive exhibits play a vital role in communicating the essence of particle physics research.
Online platforms and social media further amplify this reach, making the latest findings and ongoing research accessible to a wider audience.
Examples of Public Engagement
CERN actively engages the public through various channels. The CERN website, for example, provides comprehensive information about the LHC, its experiments, and the scientists involved. Interactive exhibits at CERN allow visitors to explore particle physics concepts in a hands-on manner. Public lectures and seminars, often featuring leading scientists, offer a direct opportunity to connect with the research.
The LHC has inspired a range of educational materials, from school programs to online resources, demonstrating the lasting impact of this cutting-edge research. These initiatives successfully convey the excitement and wonder of scientific discovery to a broader audience.
Importance of Clear Communication
Precise and engaging communication is paramount to effectively conveying the scientific significance of LHC experiments to the public. The language used must be understandable to a non-scientific audience without sacrificing the core scientific principles. Using relatable analogies and visual aids can significantly enhance comprehension. Open dialogue with journalists and the media ensures accurate reporting and broad dissemination of information.
Comparison of Public Engagement Strategies
Institution | Public Engagement Strategy |
---|---|
CERN | Extensive website with interactive elements, public lectures, educational programs, and social media presence. |
SLAC National Accelerator Laboratory | Interactive exhibits, public tours, educational outreach programs, and online resources focusing on the impacts of accelerator science on daily life. |
Fermilab | Public lectures, outreach events at schools and community centers, and online resources aimed at explaining complex scientific concepts in simple terms. |
This table provides a basic comparison of public engagement strategies employed by various particle physics research institutions. Each institution adapts its strategies to suit its specific resources and target audience, reflecting the importance of tailoring engagement efforts to maximize impact. Effective public communication remains a critical component of ensuring that the scientific community remains connected with and understood by the general public.
Last Recap
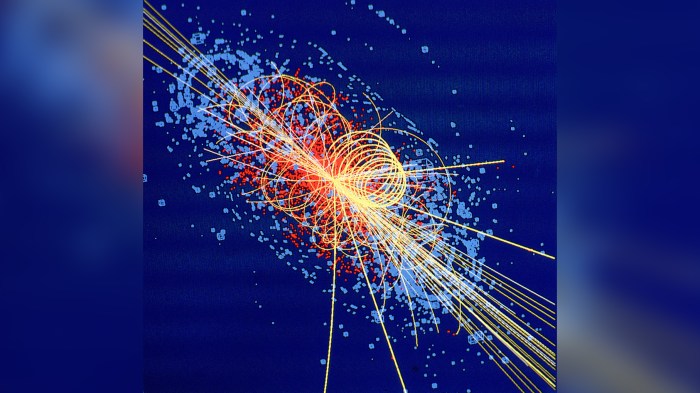
In conclusion, the Large Hadron Collider’s shattering of the energy record represents a pivotal moment in scientific history. This groundbreaking achievement, fueled by decades of research and innovation, not only expands our knowledge of the universe but also inspires future generations of scientists to push the boundaries of exploration. The implications of this record-breaking feat are vast, potentially revolutionizing our understanding of fundamental physics and opening doors to entirely new realms of discovery.